Ultrafast new camera can record light waves at 1 trillion frames per second
A new camera can take up to 1 trillion pictures per second, focusing on the previously elusive: capturing the invisible
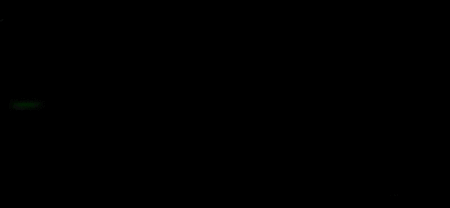
A pulse of laser light travels through a crystal in slow motion, as captured by a new ultrafast photography technology. (CREDIT: Caltech)
Over a year ago, Lihong Wang, a professor at Caltech, revolutionized imaging with a camera capable of snapping 10 trillion frames per second. This speed allowed it to capture light itself in slow motion. However, speed alone isn't always enough. Even the fastest camera can't record what it can't see.
To tackle this, Wang, Bren Professor of Medical Engineering and Electrical Engineering, has developed a new camera that can take up to 1 trillion pictures per second, focusing on the previously elusive: transparent objects. This innovation, detailed in Science Advances, opens new frontiers in capturing the invisible.
Wang’s latest invention, phase-sensitive compressed ultrafast photography (pCUP), can photograph transparent materials and fleeting phenomena like shockwaves and potentially even neural signals. This new imaging system merges high-speed photography with a century-old technique called phase-contrast microscopy, invented by Dutch physicist Frits Zernike.
Phase-contrast microscopy leverages the varying speeds of light as it travels through different materials. For instance, when light passes through glass, it slows down and then speeds up as it exits. This change in speed affects the light wave's timing. By using specific optical adjustments, scientists can distinguish light that passed through glass from light that didn’t, making the transparent material more visible.
Wang’s breakthrough adapts this principle to achieve extremely fast imaging, enabling the visualization of ultrafast events in transparent substances. “What we’ve done is to adapt standard phase-contrast microscopy so that it provides very fast imaging, allowing us to image ultrafast phenomena in transparent materials,” Wang explains.
The fast-imaging component of the system, known as lossless encoding compressed ultrafast technology (LLE-CUP), stands out in its approach. Unlike typical ultrafast video technologies that record multiple images sequentially and require repeating events, LLE-CUP captures everything in a single shot.
This single-shot method is crucial because it can capture incredibly rapid motions, such as light pulses, which would be missed by conventional cameras due to their slower imaging speed.
In their research, Wang and his team demonstrated the power of pCUP by capturing the spread of a shockwave in water and the journey of a laser pulse through a crystalline material. These examples underscore the potential of pCUP to visualize processes that are otherwise invisible.
Though still in its early stages, pCUP holds promise across various scientific fields, including physics, biology, and chemistry. Wang envisions its application in neuroscience, particularly in observing the slight expansion of nerve fibers as signals pass through them. “If we have a network of neurons, maybe we can see their communication in real time,” he says. The technology could also visualize how a flame front spreads in a combustion chamber, given that phase contrast changes with temperature.
Other applications for pCUP
Phase-sensitive compressed ultrafast photography (pCUP) has numerous real-world applications across various scientific and industrial fields:
- Biomedical Imaging:
- Cellular and Molecular Dynamics: pCUP can capture rapid processes in living cells, such as protein folding, molecular interactions, and intracellular signaling, providing insights into fundamental biological mechanisms.
- Neuroimaging: It can be used to study fast neuronal activities and signal transmission in the brain, aiding in the understanding of neurological diseases and the development of new treatments.
- Chemical Reactions:
- Reaction Kinetics: pCUP enables the observation of ultrafast chemical reactions and intermediate states, crucial for developing new catalysts and understanding reaction mechanisms.
- Photochemical Processes: It can capture the dynamics of light-induced chemical reactions, aiding in the design of more efficient solar energy conversion systems and photodynamic therapies.
- Materials Science:
- Phase Transitions: pCUP can observe rapid phase transitions in materials, providing insights into the development of advanced materials with tailored properties for electronics, photonics, and energy storage.
- Strain and Stress Analysis: It can be used to study the behavior of materials under high strain rates, informing the design of materials for aerospace, automotive, and civil engineering applications.
- Optics and Photonics:
- Laser Dynamics: pCUP can capture the dynamics of laser pulses and interactions with matter, useful for optimizing laser systems in communications, manufacturing, and medical applications.
- Nonlinear Optical Phenomena: It enables the study of ultrafast nonlinear optical processes, which are critical for developing advanced optical devices and communication technologies.
- Fluid Dynamics:
- Turbulence and Shock Waves: pCUP can visualize fast fluid dynamics phenomena, such as turbulence and shock waves, improving the understanding of aerodynamic and hydrodynamic systems.
- Cavitation: It can capture the formation and collapse of cavitation bubbles, relevant for improving industrial processes and understanding the mechanisms of cavitation-induced damage.
- Plasma Physics:
- Plasma Dynamics: pCUP can observe the behavior of plasmas in fusion experiments, astrophysical phenomena, and industrial applications, contributing to the development of controlled nuclear fusion and advanced plasma technologies.
- Security and Defense:
- Explosive Dynamics: It can capture the rapid events involved in explosions, aiding in the development of better protective measures and forensic analysis of explosive events.
- Projectile Motion: pCUP can track the high-speed motion of projectiles, informing the design of more effective defense systems and improving ballistics research.
The groundbreaking paper titled “Picosecond-resolution phase-sensitive imaging of transparent objects in a single shot” lists co-authors Taewoo Kim, a postdoctoral scholar in medical engineering, and former Caltech researchers Jinyang Liang and Liren Zhu. This research was supported by the National Institutes of Health.
Wang's affiliation with the Tianqiao and Chrissy Chen Institute for Neuroscience at Caltech underscores his commitment to advancing our understanding of the brain. As the development of pCUP continues, its ability to reveal the unseen could usher in new scientific discoveries and applications, making the invisible, visible.
Note: Materials provided above by the The Brighter Side of News. Content may be edited for style and length.
Like these kind of feel good stories? Get the Brighter Side of News' newsletter.
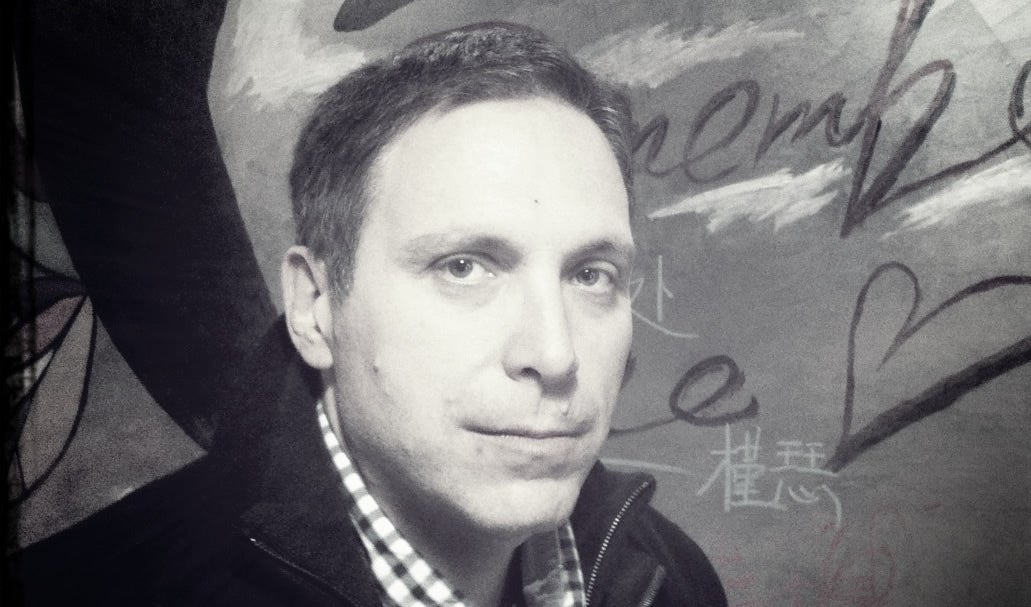