Strange new particle only has mass when moving in one direction
For the first time, scientists have caught a glimpse of a strange and elusive creature in the world of quantum physics—a semi-Dirac fermion.
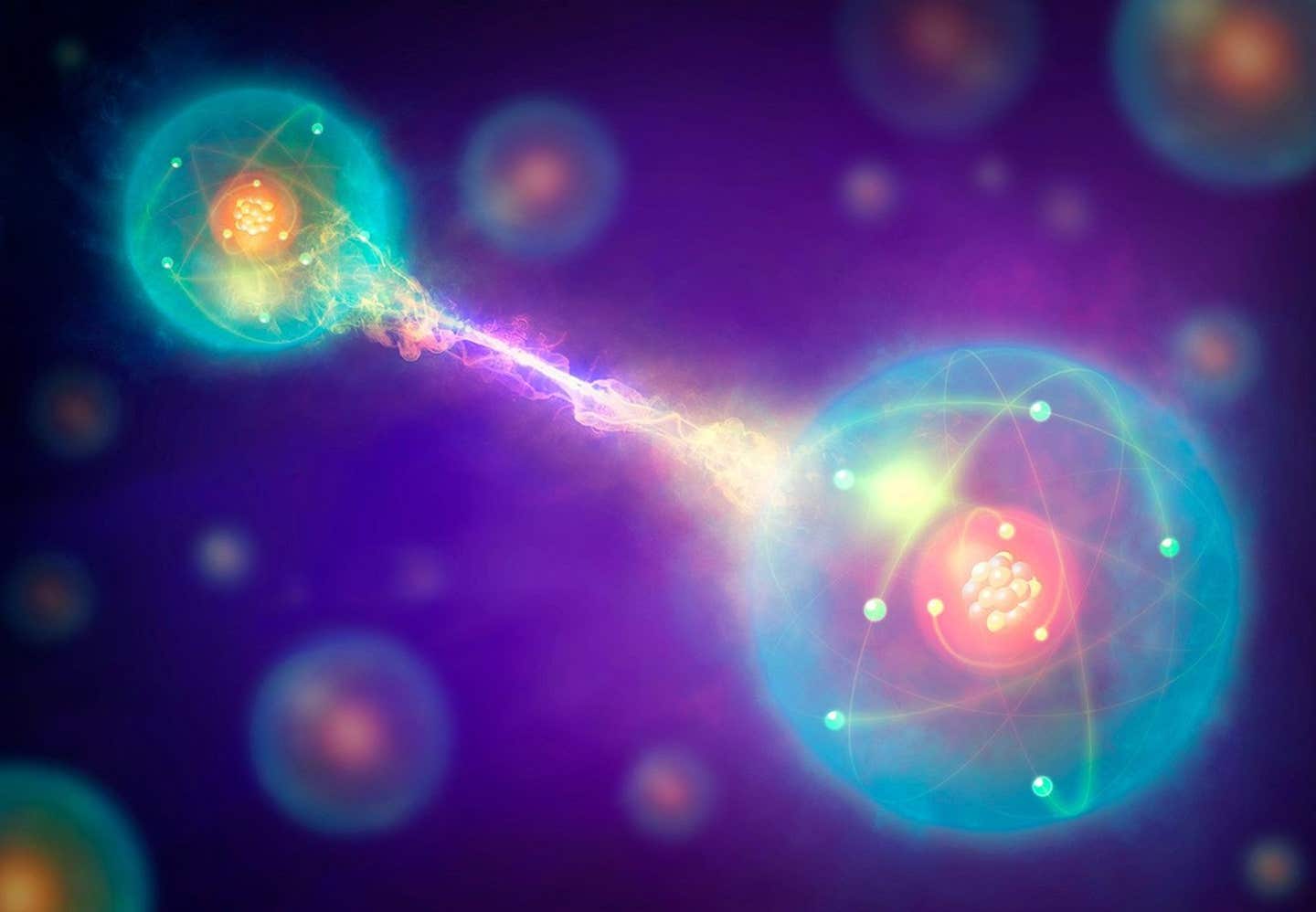
Quasiparticles like semi-dirac fermions are not actual particles, but they arise from the collective behavior of many electrons in solid matter. (CREDIT: Getty Images)
For the first time, scientists have caught a glimpse of a strange and elusive creature in the world of quantum physics—a semi-Dirac fermion. This unusual quasiparticle behaves as if it has no mass in one direction, yet acts massive in another. The discovery happened inside a crystal of the semi-metal ZrSiS and was recently reported in Physical Review X by a research team from Penn State and Columbia University.
“We weren’t even looking for a semi-Dirac fermion,” said Yinming Shao, a physics professor and lead author on the study. “We were seeing signatures we didn’t understand — and it turns out we had made the first observation of these wild quasiparticles that sometimes move like they have mass and sometimes move like they have none.”
What Are Quasiparticles
Quasiparticles like these are not actual particles, but they arise from the collective behavior of many electrons in solid matter. As electrons interact with each other and with atoms in the material, they can act as if they are entirely new entities. In this case, the emergent semi-Dirac fermion is massless in one direction but carries mass in another—a result of the material’s unusual electronic structure.
Though predicted over 16 years ago, semi-Dirac fermions had remained a theoretical oddity until now. Physicists long believed that specific materials might support these hybrids of mass and masslessness, but direct proof had eluded them. That is, until this surprising find.
The key to unlocking the quasiparticle’s behavior came through a technique called magneto-optical spectroscopy. This approach involves bombarding a material with infrared light while it sits in a strong magnetic field. In ZrSiS, the method revealed unexpected behavior in the way electrons absorb and respond to that light.
Unlocking The Quasiparticle’s Behavior
To make this happen, the team used a lab that boasts the world’s strongest continuous magnetic field—the National High Magnetic Field Laboratory in Florida. Its hybrid magnet produces a field more than 900,000 times stronger than Earth’s own magnetic pull. This extraordinary strength was essential to revealing the subtle quantum effects hidden inside the crystal.
Related Stories
To sharpen their view even further, the researchers cooled the ZrSiS samples to -452 degrees Fahrenheit, close to absolute zero. These ultra-low temperatures removed thermal noise, letting the scientists isolate and observe the material’s raw quantum behavior under the extreme magnetic conditions.
Shao explained that when a magnetic field is applied, electrons in a material don’t move freely—they settle into distinct energy levels called Landau levels. The spacing of these levels depends on the electrons’ mass and the field’s strength. It was within this spacing pattern that the signature of the semi-Dirac fermion finally came into focus.
However, the levels in ZrSiS followed an unusual pattern, aligning with a theoretical “B^(2/3) power law” that had been predicted as a signature of semi-Dirac fermions. The experiment thus provided definitive evidence of these quasiparticles.
Quasiparticle Movement
Theoretical physicists partnered with the experimental team to model ZrSiS’s electronic structure. They found that the material’s electrons moved along specific pathways, or “tracks,” where their behavior shifted depending on direction.
“Imagine the particle is a tiny train confined to a network of tracks,” Shao said. “At certain intersections, the train switches from a fast track to a slower one, experiencing resistance and gaining mass.” This directional dependence is the defining feature of semi-Dirac fermions.
The observed behavior of electrons in ZrSiS reflects the material’s underlying structure, which includes planar nodal squares connected by vertical nodal lines. These features form a chainlike configuration in momentum space, creating conditions for semi-Dirac fermions to emerge.
Ab initio calculations confirmed that these quasiparticles originated at specific crossing points within the nodal lines. Near these points, electrons exhibited linear energy dispersion in one direction and quadratic dispersion in the perpendicular direction, matching the theoretical predictions for semi-Dirac behavior.
Potential Application of This Discovery
The potential applications of this discovery extend far beyond basic physics. Materials exhibiting semi-Dirac behavior could revolutionize technologies that rely on advanced electronic properties, such as batteries, sensors, and quantum devices.
Shao highlighted that ZrSiS, like graphite, has a layered structure, making it a candidate for further refinement. By isolating single layers, researchers could precisely control the material’s properties, much like graphene.
“It is a layered material, which means once we can figure out how to have a single-layer cut of this compound, we can harness the power of semi-Dirac fermions,” Shao said. “The most thrilling part of this experiment is that the data cannot be fully explained yet. There are many unsolved mysteries in what we observed, so that is what we are working to understand.”
This pioneering research involved collaboration among experts from Penn State, Columbia University, and other institutions, including Temple University, Florida State University, and Radboud University in the Netherlands. The U.S. National Science Foundation, the Department of Energy, and the Simons Foundation provided funding for the project.
The discovery of semi-Dirac fermions marks a significant advance in condensed matter physics. While theoretical studies had long predicted their existence, their experimental realization within ZrSiS opens new avenues for exploring topological and correlated phases of matter.
It also underscores the power of collaborative, interdisciplinary approaches in uncovering fundamental quantum phenomena.
Note: Materials provided above by The Brighter Side of News. Content may be edited for style and length.
Like these kind of feel good stories? Get The Brighter Side of News' newsletter.
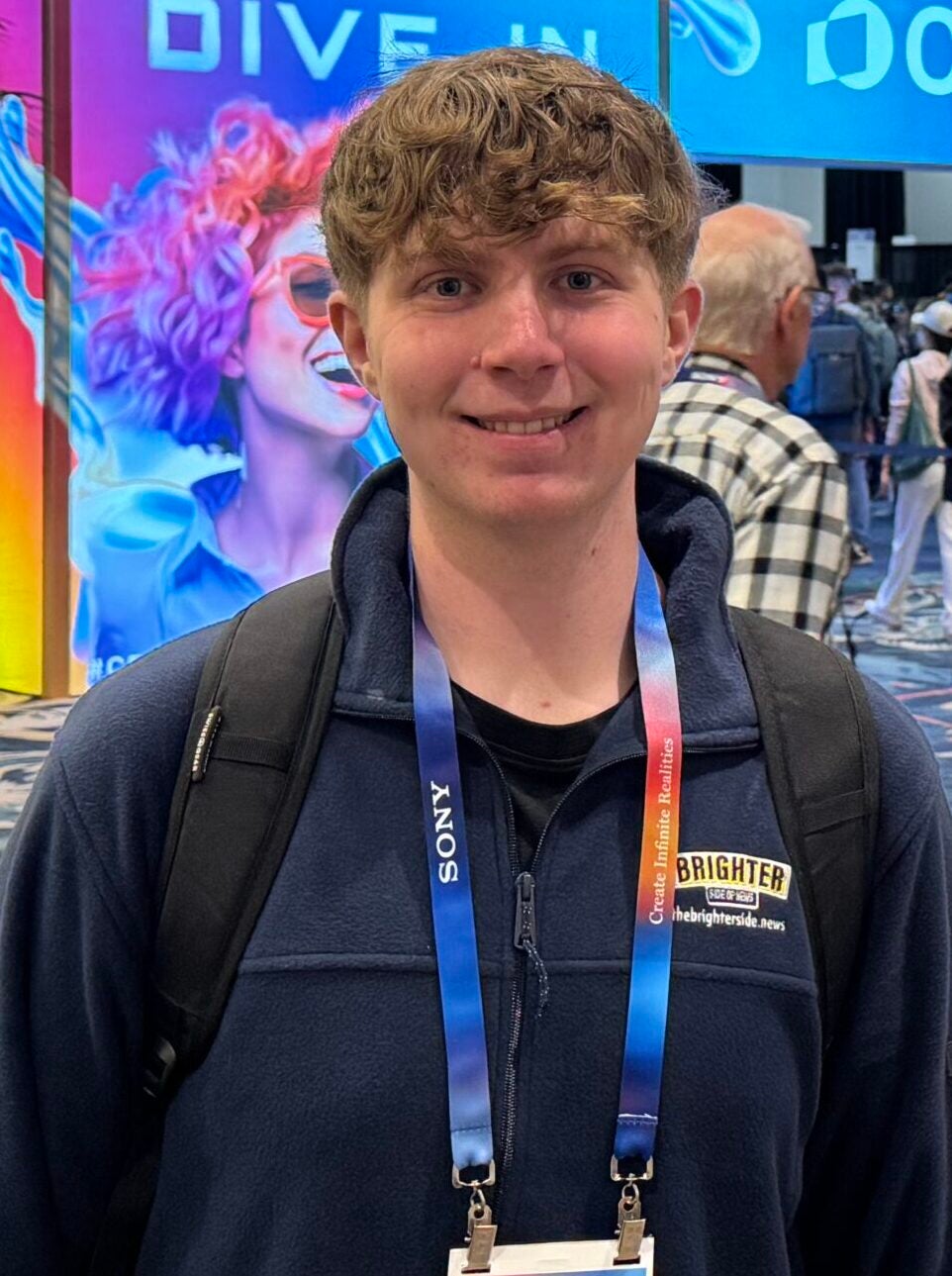
Joshua Shavit
Science & Technology Writer | AI and Robotics Reporter
Joshua Shavit is a Los Angeles-based science and technology writer with a passion for exploring the breakthroughs shaping the future. As a contributor to The Brighter Side of News, he focuses on positive and transformative advancements in AI, technology, physics, engineering, robotics and space science. Joshua is currently working towards a Bachelor of Science in Business Administration at the University of California, Berkeley. He combines his academic background with a talent for storytelling, making complex scientific discoveries engaging and accessible. His work highlights the innovators behind the ideas, bringing readers closer to the people driving progress.