Scientists use the Lorentz force to stop light in its tracks
A simple deformation of a two-dimensional photonic crystal can bring light waves to a complete halt—a feat that could reshape the future of nanophotonic technology.
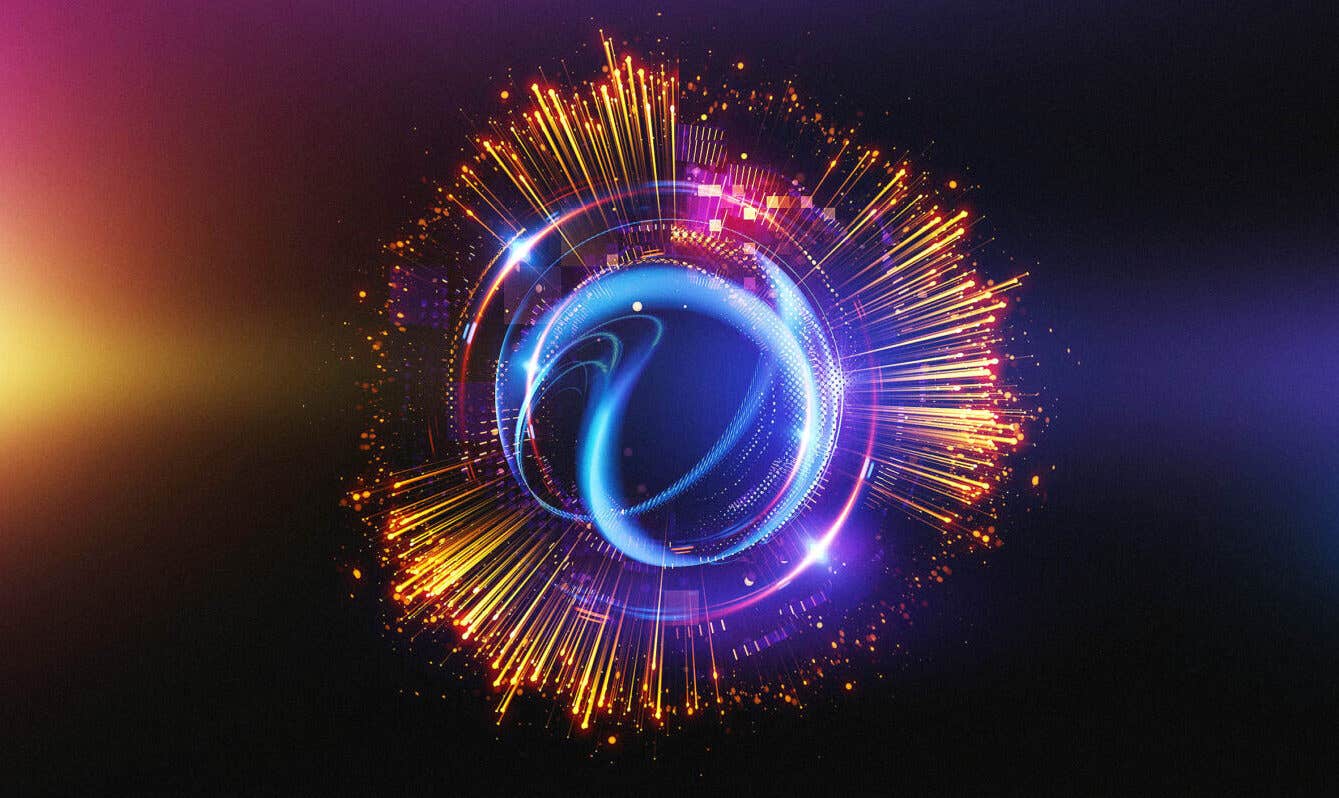
Scientists have developed an innovative method to halt light waves entirely by altering the structure of a two-dimensional photonic crystal. (CREDIT: CC BY-SA 4.0)
Scientists from AMOLF, in partnership with Delft University of Technology, have pioneered a groundbreaking method for controlling light at the nanoscale.
Their latest research, featured in Nature Photonics, reveals how a simple deformation of a two-dimensional photonic crystal can bring light waves to a complete halt—a feat that could reshape the future of nanophotonic technology.
This achievement, independently replicated by researchers at Pennsylvania State University and published in the same journal, represents a major leap forward in photonics. The ability to manipulate light so precisely could pave the way for a new generation of ultra-efficient optical devices.
On a microscopic level, controlling the movement of light within materials is fundamental to developing high-performance nanophotonic chips. These tiny components serve as the backbone of emerging optical technologies.
In traditional electronics, scientists regulate the flow of electrons using magnetic fields, but light—made up of uncharged photons—does not respond to such forces in the same way.
For electrons, the influence of a magnetic field is governed by the Lorentz force, which alters their trajectory and enables precise control. Without an electric charge, photons lack this built-in mechanism, requiring researchers to explore alternative methods to manipulate their movement.
The Photonic Forces group at AMOLF has been investigating ways to create a "magnetic field-like" effect for photons. By engineering materials with unique optical properties, they aim to direct and confine light in ways previously thought impossible.
Lead researcher Ewold Verhagen explains that their work was inspired by electron behavior in condensed matter physics. "In a conductor, electrons move freely, but a strong magnetic field can disrupt this motion, forcing them into circular paths. This restriction prevents conduction and locks electrons into specific energy levels known as Landau levels," he says. These discrete energy states are a fundamental feature of electrons under a magnetic field's influence.
A particularly intriguing parallel exists in graphene, a single layer of carbon atoms arranged in a honeycomb pattern. "While graphene is naturally an excellent conductor, stretching it like a rubber band alters its atomic structure, effectively turning it into an insulator," Verhagen explains. "This mechanical strain forces electrons into discrete energy states, mimicking the behavior seen in a magnetic field."
With this in mind, the researchers wondered whether a similar approach could be applied to photons. By carefully deforming a photonic crystal, they discovered a way to control light in much the same manner, offering a revolutionary new tool for shaping the behavior of photons in nanoscale devices.
Related Stories
Photonic Crystals: A Platform for Light Manipulation
Through a collaboration with Kobus Kuipers of Delft University of Technology, Verhagen's group successfully demonstrated an analogous effect for light within a photonic crystal.
"A photonic crystal is typically a two-dimensional array of holes etched into a silicon layer," explains René Barczyk, the first author and recent PhD graduate from AMOLF. "Light can propagate freely through this material, similar to how electrons move in graphene. However, by meticulously disrupting this regularity, we can deform the crystal structure and confine the photons. This essentially creates Landau levels for light."
Within these Landau levels, light waves cease to propagate; they become stationary within the crystal. The researchers confirmed this phenomenon, demonstrating that the deformed crystal has a similar effect on photons as a magnetic field on electrons.
Verhagen elaborates, "By manipulating the deformation pattern, we can even generate various types of effective magnetic fields within the same material. This allows for selective movement of light in specific regions of the crystal, offering new avenues for controlling light on a chip."
Simultaneous Discoveries Pave the Way for Future Applications
The research by Verhagen's group was inspired by theoretical predictions from researchers at Pennsylvania State University and Columbia University.
Verhagen recounts, "During our initial measurements, I had the opportunity to discuss our work with one of the authors from the other study. Upon learning of their efforts to achieve the same effect experimentally, we decided to collaborate and submit our findings simultaneously. While the approaches differed slightly, both teams successfully stopped light waves and observed Landau levels by deforming a two-dimensional photonic crystal."
This achievement brings significant potential for on-chip applications, as Verhagen emphasizes, "The ability to confine and halt light at the nanoscale can significantly enhance its intensity. This wouldn't be limited to a single location but achievable across the entire crystal surface. Such concentrated light is essential for nanophotonic devices, paving the way for efficient lasers and quantum light sources."
The successful manipulation of light using a "magnetic field-like" approach in photonic crystals opens exciting possibilities for the future of nanophotonics.
With further research, this technology has the potential to revolutionize various fields, from optical communication to quantum computing.
Note: Materials provided above by The Brighter Side of News. Content may be edited for style and length.
Like these kind of feel good stories? Get The Brighter Side of News' newsletter.
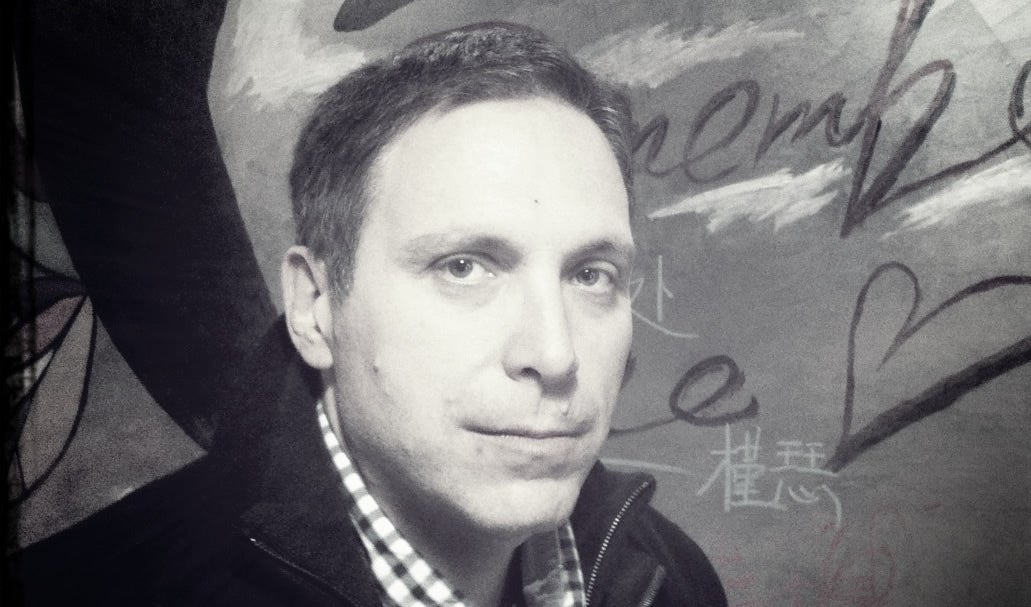
Joseph Shavit
Head Science News Writer | Communicating Innovation & Discovery
Based in Los Angeles, Joseph Shavit is an accomplished science journalist, head science news writer and co-founder at The Brighter Side of News, where he translates cutting-edge discoveries into compelling stories for a broad audience. With a strong background spanning science, business, product management, media leadership, and entrepreneurship, Joseph brings a unique perspective to science communication. His expertise allows him to uncover the intersection of technological advancements and market potential, shedding light on how groundbreaking research evolves into transformative products and industries.