Scientists use evolution to bioengineer drugs and sustainable energy
Engineered photosynthetic hybrids merge yeast and cyanobacteria to produce valuable compounds and recycle CO2, transforming biotechnological sustainability
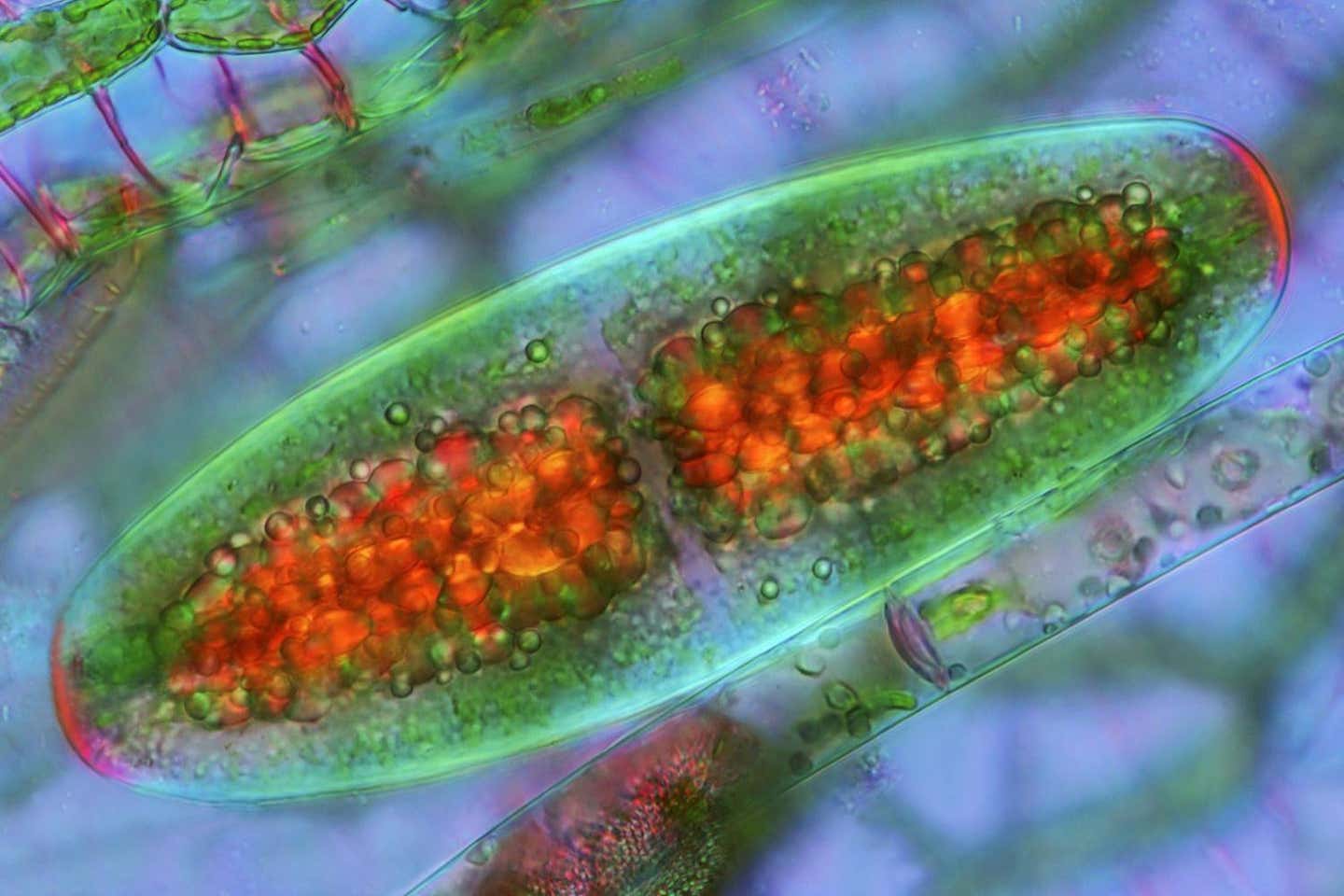
Researchers have created yeast-cyanobacteria hybrids that utilize CO2 for growth, paving the way for carbon-neutral biotechnological solutions and sustainable production of fuels and pharmaceuticals. (CREDIT: Marek Mis/Science Photo Library/Getty Images)
The quest to transform biology into a tool for sustainable production is making strides in the field of metabolic engineering. Researchers are designing innovative ways to harness the potential of photosynthetic organisms and genetic modifications to produce valuable chemicals and reduce reliance on traditional carbon sources.
At the heart of this advancement lies the development of model organisms that can assimilate carbon dioxide (CO2) as a primary feedstock.
Traditionally, bacteria like Escherichia coli and yeasts such as Saccharomyces cerevisiae have relied on external carbon sources, such as glucose and glycerol, to thrive. However, the growing demand for carbon-neutral processes has led to efforts to reengineer these organisms into autotrophs that can directly utilize CO2.
Metabolic engineering techniques have been pivotal in converting heterotrophic organisms into autotrophic or semi-autotrophic systems. By introducing CO2-fixation pathways, such as the Calvin–Benson–Bassham (CBB) cycle, scientists have enabled organisms to channel CO2 into their central metabolic processes. Enzymes like RuBisCO and phosphoribulokinase (PRK) play a critical role in these transformations.
One breakthrough example is the engineering of E. coli strains capable of efficient CO2 recycling. Similarly, researchers have adapted yeasts like Pichia pastoris and S. cerevisiae to grow on CO2. These engineered organisms have shown promise in producing key biochemicals, including ethanol, ethylene, and butanediol.
In other cases, cyanobacteria and microalgae have been engineered for photomixotrophic chemical production, extending the range of synthetic biology applications.
Directed Endosymbiosis: A Revolutionary Approach
Inspired by the evolutionary history of chloroplasts, researchers have pioneered a concept known as directed endosymbiosis. This approach mimics the natural process by which free-living cyanobacteria became endosymbionts in ancestral eukaryotic cells, ultimately evolving into chloroplasts.
Related Stories
“Our work is rooted in evolutionary principles,” said Angad Mehta, a chemistry professor leading the study. “Endosymbiosis gave rise to complex life forms, and now we are applying this process in the lab to engineer new possibilities.”
Mehta’s team has developed yeast-cyanobacteria hybrids where photosynthetic bacteria live symbiotically within yeast cells. These chimeric systems allow yeast to thrive without traditional carbon feedstocks. Instead, the cyanobacteria perform photosynthetic carbon assimilation, supplying sugars and ATP directly to the yeast cells.
In laboratory experiments, these engineered hybrids have demonstrated the ability to grow for several generations in CO2-rich environments, even in the absence of glucose or glycerol.
The team has also engineered metabolic pathways within these hybrids to produce valuable compounds like limonene under photosynthetic conditions. Limonene, a simple hydrocarbon commonly found in citrus fruits, serves as a precursor for fuels and pharmaceuticals.
“This proof-of-concept shows that we can bioengineer pathways in our hybrids to photosynthetically produce valuable molecules,” Mehta explained. “We aim to expand this capability to more complex compounds, such as anticancer and antimalarial drugs.”
A Path to Carbon Neutrality
The ultimate goal of this research is to create systems capable of recycling CO2 into high-value products on a commercial scale. By refining these processes, the potential exists to significantly reduce industrial carbon emissions while producing essential chemicals sustainably.
“I think it would be incredible to assure that every bit of carbon in a high-value compound comes from CO2,” Mehta said. “This could be a game-changer in recycling CO2 waste in the future.”
The team also sees an opportunity to answer fundamental questions about evolution. As they work to perfect endosymbiotic systems, they uncover insights into the processes that shaped life on Earth. “Recreating evolution in the lab is one of the best ways to understand it,” Mehta remarked.
Beyond their immediate applications, these engineered systems have broader implications. They could serve as platforms to study evolutionary adaptation and provide molecular insights into how endosymbionts transform into organelles.
This research opens new doors for synthetic biology and lays the groundwork for a future where biology addresses some of humanity's most pressing challenges.
The study, published in Nature Communications, represents a collaborative effort by researchers at the University of Illinois Urbana-Champaign.
Supported by the National Institutes of Health, the findings highlight the potential of directed endosymbiosis to revolutionize biotechnology and tackle global sustainability challenges.
Note: Materials provided above by The Brighter Side of News. Content may be edited for style and length.
Like these kind of feel good stories? Get The Brighter Side of News' newsletter.
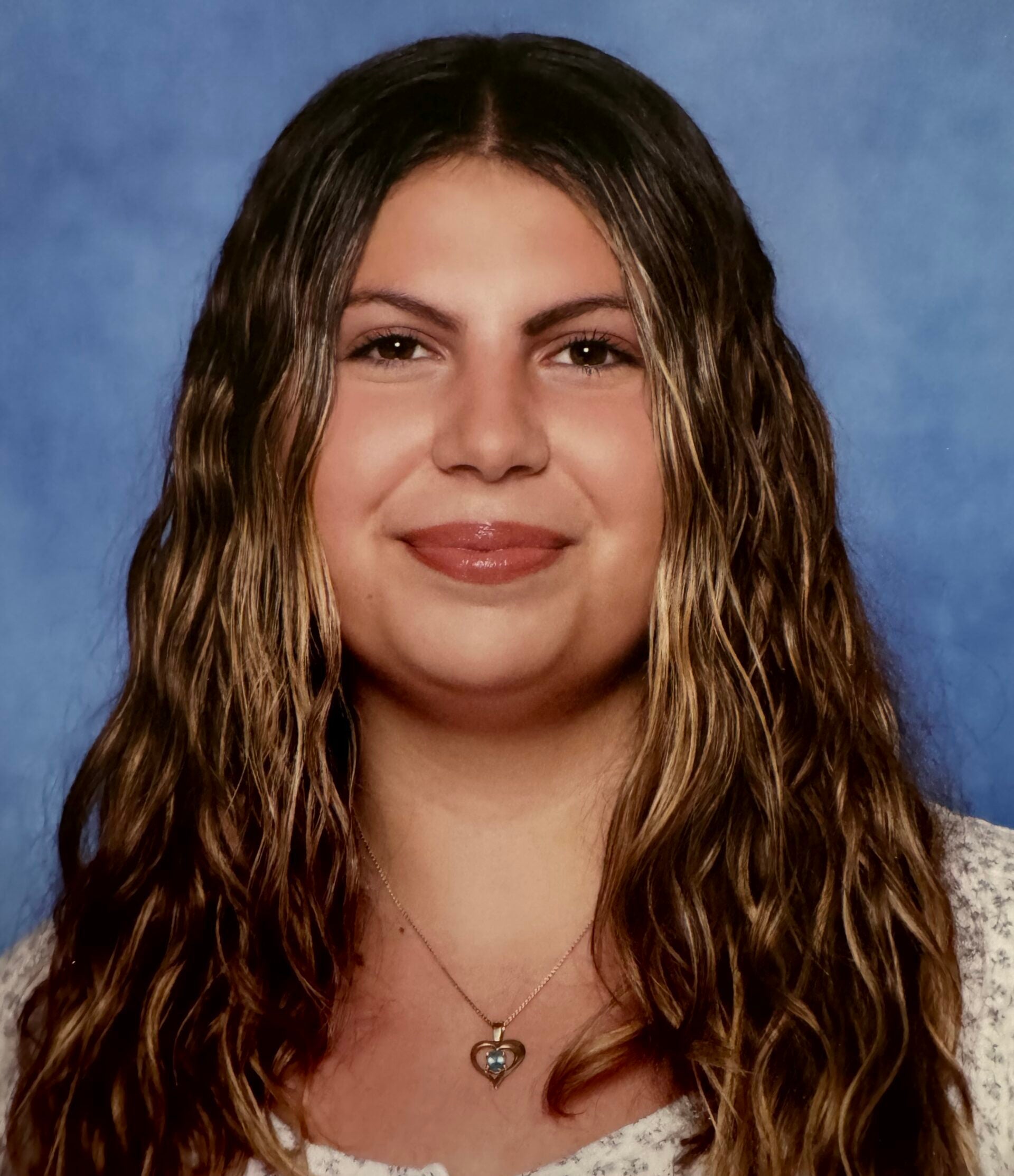
Rebecca Shavit
Science & Technology Journalist | Innovation Storyteller
Based in Los Angeles, Rebecca Shavit is a dedicated science and technology journalist who writes for The Brighter Side of News, an online publication committed to highlighting positive and transformative stories from around the world. With a passion for uncovering groundbreaking discoveries and innovations, she brings to light the scientific advancements shaping a better future. Her reporting spans a wide range of topics, from cutting-edge medical breakthroughs and artificial intelligence to green technology and space exploration. With a keen ability to translate complex concepts into engaging and accessible stories, she makes science and innovation relatable to a broad audience.