Scientists unite Einstein’s relativity and quantum mechanics through Schrödinger’s cat equation
Quantum mechanics operates in a bizarre world where particles can exist in multiple states simultaneously, a concept known as superposition.
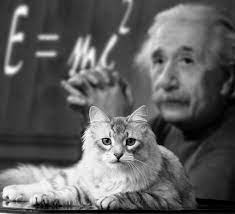
Recent study introduces modifications to the fundamental Schrödinger equation, the cornerstone of quantum mechanics. (CREDIT: CC BY-SA 4.0)
The intricate relationship between quantum mechanics and classical physics has long puzzled scientists. Quantum mechanics operates in a bizarre world where particles can exist in multiple states simultaneously, a concept known as superposition. However, this principle appears to break down in the macroscopic realm.
Planets, stars, and even the universe itself don't exhibit such superpositions, creating a significant challenge in understanding how the universe transitions from quantum to classical behavior.
At the heart of this enigma is the question: how does the universe, if fundamentally quantum, adhere to classical laws like general relativity? This puzzle has led to groundbreaking work by researchers such as Matteo Carlesso and his colleagues at the University of Trieste.
Their recent study introduces modifications to the fundamental Schrödinger equation, the cornerstone of quantum mechanics, to bridge this conceptual divide.
The Measurement Problem and Superposition
The quantum measurement problem arises from the inability to determine what qualifies as a measurer in quantum mechanics. John Bell famously questioned, “What exactly qualifies some physical systems to play the role of measurer?”
In quantum theory, particles exist in a superposition of states until a measurement collapses them into a definite state. Schrödinger’s thought experiment about a cat that is simultaneously alive and dead exemplifies this idea. Yet, this framework faces challenges when applied to macroscopic systems or the universe as a whole.
The classical behavior of the universe is evident in phenomena like the Cosmic Microwave Background (CMB), a relic signal from the early universe. While the CMB’s quantum origins are supported by frameworks like inflationary cosmology, its observable properties align with classical predictions. This raises the critical question: how did the universe transition from a quantum to a classical state?
Related Stories
Carlesso’s team addresses this transition through models of spontaneous wavefunction collapse. These models modify the Schrödinger equation by introducing nonlinear and stochastic terms, which cause the collapse of quantum superpositions without requiring external observers or environments.
This mechanism becomes increasingly effective as systems grow larger, providing a natural explanation for why macroscopic systems exhibit classical behavior.
“The question is whether the Universe, lacking an external environment, can be in a superposition,” Carlesso explains. Observations suggest otherwise, showing adherence to classical laws. To resolve this, the researchers propose self-induced collapse mechanisms where systems interact with themselves, causing spontaneous localization into definite states.
“Without external influence, any system spontaneously collapses into a specific state,” Carlesso clarifies. This approach removes the distinction between measured systems and measuring devices, treating all entities equally under the collapse mechanism.
Quantum Cosmology and Space-Time
In their study, the researchers focus on the quantumness of space-time rather than matter fields. At the universe’s earliest stages, it might have existed in a superposition of different geometries. Through the proposed collapse mechanism, the universe transitioned into a single, classical geometry, enabling the observable classical dynamics that align with general relativity.
The researchers restrict their analysis to a flat, maximally symmetric, homogeneous, and isotropic Friedmann-Lemaître-Robertson-Walker (FLRW) universe. This model adheres to the cosmological principle, describing a universe where space-time is foliated into hypersurfaces connected by a time-like direction.
By incorporating spontaneous collapse models, Carlesso’s team bridges the gap between a quantum cosmological model in its infancy and a classical universe observable today.
“Our model describes a quantum universe that eventually collapses, becoming effectively classical,” Carlesso elaborates. This explanation does not predict deviations from classical cosmology after the CMB photon emission but provides a robust framework for understanding earlier stages of the universe.
Experimental Challenges and Prospects
Despite its theoretical elegance, testing the predictions of spontaneous collapse models poses significant challenges. These models suggest subtle deviations from conventional quantum mechanics at the level of atoms and molecules. However, such deviations are incredibly small, requiring highly sensitive experimental setups.
Carlesso and his colleagues are actively collaborating with experimental physicists to devise innovative methods for testing their model. These efforts aim to validate or refute the theory, pushing the boundaries of quantum mechanics and cosmology. If successful, these experiments could offer new insights into the fundamental workings of the universe and its transition from quantum chaos to classical order.
This groundbreaking study offers a fresh perspective on the quantum-to-classical transition, a pivotal issue in modern physics. By modifying the Schrödinger equation and introducing the concept of self-induced collapse, Carlesso’s team has paved the way for a deeper understanding of the universe’s evolution.
While challenges remain in experimental verification, their work represents a significant step toward reconciling the quantum and classical worlds.
Note: Materials provided above by The Brighter Side of News. Content may be edited for style and length.
Like these kind of feel good stories? Get The Brighter Side of News' newsletter.
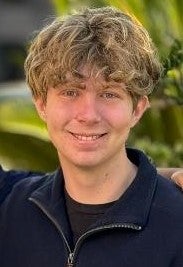