Scientists make major breakthrough in high-energy particle detection
Superconducting nanowire single-photon detectors, once limited to photon detection, now show promise for high-energy proton detection.
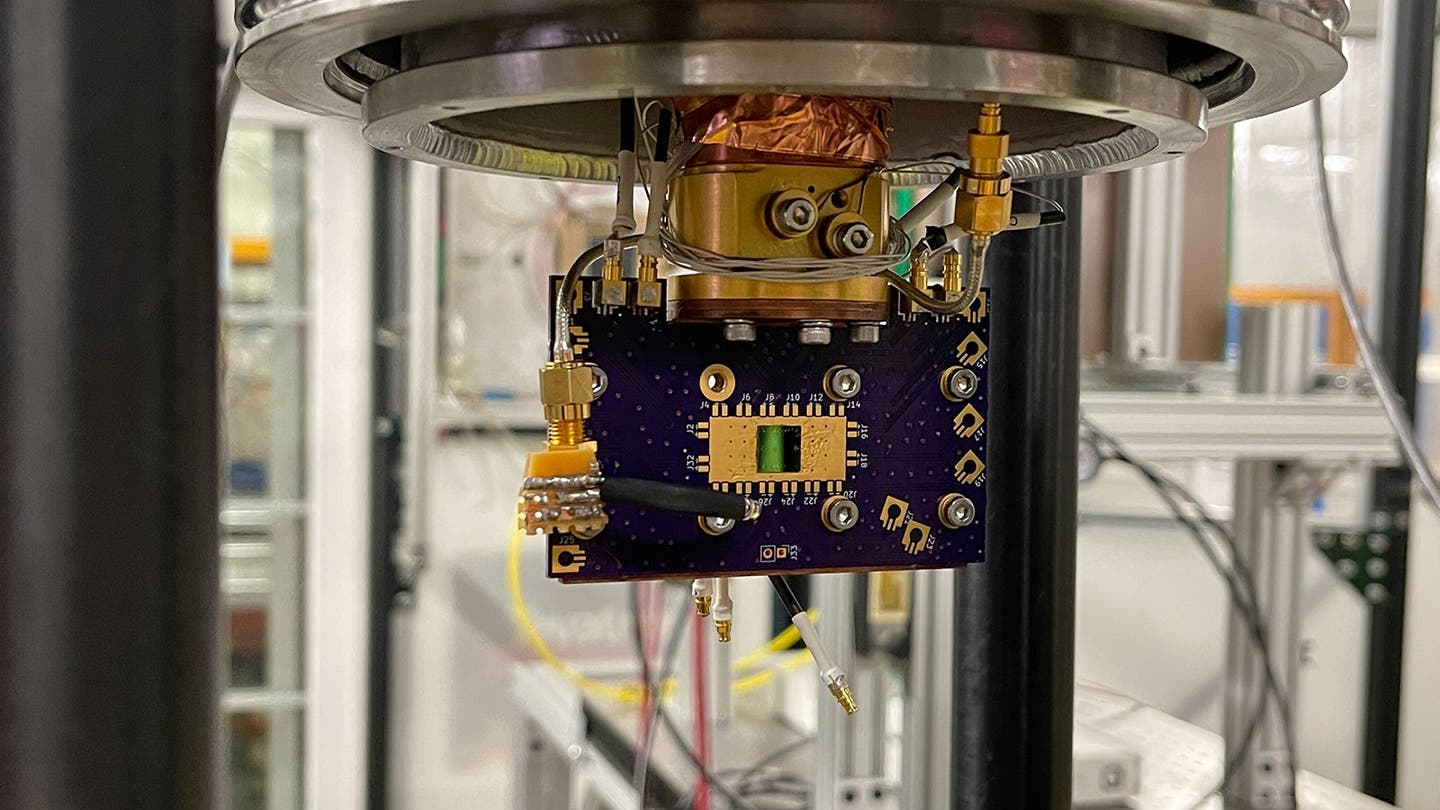
Close-up view of a SNSPD mounted on a printed circuit board inside the cryostat at the Fermilab Test Beam Facility. This device was used in the first successful demonstration of high-energy proton detection using SNSPDs. (CREDIT: Sangbaek Lee/Argonne National Laboratory)
Detecting high-energy particles is essential for exploring the fundamental structure of matter. Particle accelerators propel charged particles close to the speed of light, smashing them into targets to reveal their properties.
Traditional detectors struggle with sensitivity and precision at extreme scales, limiting their use in advanced physics experiments. However, a new approach using superconducting nanowire single-photon detectors (SNSPDs) has demonstrated an unexpected ability to detect high-energy protons with remarkable accuracy.
Scientists at the U.S. Department of Energy’s (DOE) Argonne National Laboratory have successfully adapted SNSPDs—previously used for detecting photons—to identify charged particles. This development opens new possibilities for nuclear and particle physics, particularly for experiments requiring precise spatial and timing resolution.
“This was a first-of-its-kind use of the technology,” said Argonne physicist Whitney Armstrong. “This step was critical to demonstrate that the technology works the way we want it to because it is typically geared toward photons. It was a key demonstration for future high-impact applications.”
Transforming Photon Sensors into Particle Detectors
SNSPDs work by absorbing individual photons, generating small electrical signals when superconducting nanowires are disturbed. These sensors operate at extremely low temperatures, allowing them to measure single photons with exceptional precision. Such capabilities are essential for applications in quantum cryptography, optical sensing, and quantum computing.
The research team theorized that SNSPDs could also detect charged particles, such as protons. To test this idea, they built detectors with different nanowire widths and exposed them to a 120 GeV proton beam at Fermilab, a leading facility for high-energy particle studies.
The results confirmed that SNSPDs can detect protons with high efficiency. The optimal wire width for this application was around 250 nanometers—far smaller than the width of a human hair, which measures approximately 100,000 nanometers. Wires thinner than 400 nanometers performed particularly well, demonstrating a strong capability for sensing high-energy particles.
“This was a successful technology transfer between quantum sciences, for photon detection, into experimental nuclear physics,” said Argonne physicist Tomas Polakovic. “We took the photon-sensing device and made slight changes to make it work better in magnetic fields and for particles. And behold, we saw the particles exactly as we expected.”
Related Stories
Unlocking New Possibilities for High-Energy Physics
One of the most promising applications of SNSPDs is their use in next-generation particle accelerators. The Electron-Ion Collider (EIC), currently under development at DOE’s Brookhaven National Laboratory, will use high-energy collisions to study the internal structure of protons and neutrons. The collider will require extremely sensitive detectors to track particles with high precision.
SNSPDs could play a crucial role in this facility. Their ability to operate in strong magnetic fields makes them ideal for use in the superconducting magnets of the EIC. Since the protons tested at Fermilab had energy levels within the EIC’s range, the study provides a foundation for using SNSPDs in future collider experiments.
“The proton energy range that we tested at Fermilab is right in the middle of the span of the ion’s energy range that we will detect at EIC, so these tests were well-suited,” said Sangbaek Lee, a physics postdoctoral appointee at Argonne.
SNSPDs' compact size, precise timing, and resilience under extreme conditions make them a strong alternative to traditional semiconductor detectors. Unlike conventional particle sensors, which can be bulky and affected by beamline magnets, SNSPDs can be integrated into the cryogenic environment of superconducting magnets, maximizing their effectiveness in collider experiments.
Expanding the Scope of Particle Detection
This research marks a major step toward improving high-energy particle detection. SNSPDs' successful adaptation from photon detection to proton sensing demonstrates their versatility, potentially benefiting a wide range of scientific fields.
The study was made possible through advanced nanofabrication techniques at Argonne’s Center for Nanoscale Materials. Researchers used a specialized Reactive Ion Etching tool to create the nanowires, optimizing their dimensions for proton detection. The findings were published in Nuclear Instruments and Methods in Physics Research Section A, with funding provided by the DOE Office of Science, Office of Nuclear Physics.
The potential applications extend beyond physics. The ability to detect single particles with high precision could lead to innovations in medical imaging, materials science, and secure communication. With further refinement, SNSPDs could become a standard tool for next-generation particle experiments, pushing the boundaries of what is possible in fundamental science.
“This work proves that SNSPDs can go beyond their original purpose,” said Armstrong. “By demonstrating their ability to detect protons, we’ve opened new doors for using this technology in high-energy physics.”
As research continues, SNSPDs may become an essential component in future accelerators and experiments, offering unprecedented sensitivity and efficiency in detecting the universe’s most fundamental particles.
Note: Materials provided above by The Brighter Side of News. Content may be edited for style and length.
Like these kind of feel good stories? Get The Brighter Side of News' newsletter.
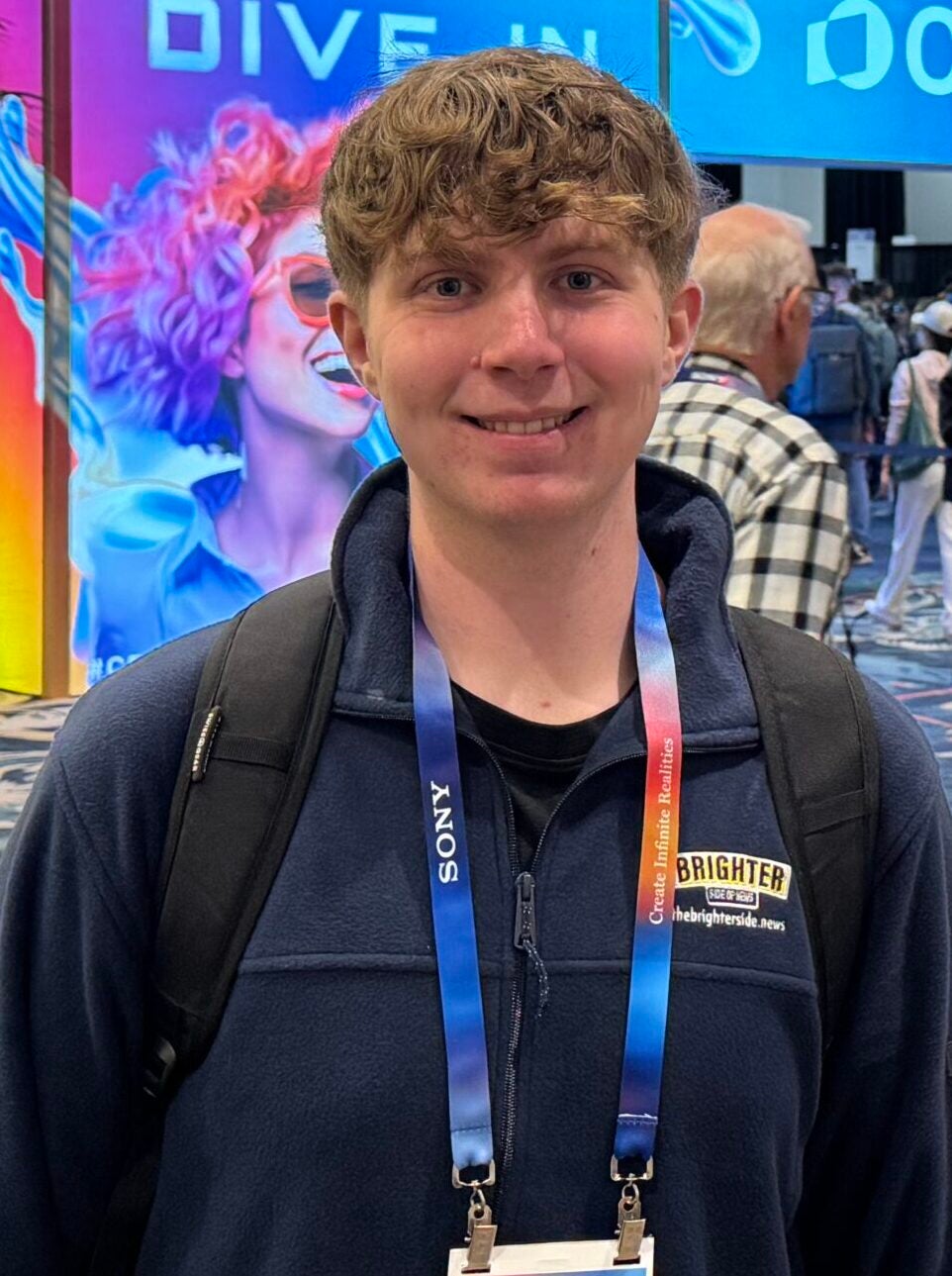
Joshua Shavit
Science & Technology Writer | AI and Robotics Reporter
Joshua Shavit is a Los Angeles-based science and technology writer with a passion for exploring the breakthroughs shaping the future. As a contributor to The Brighter Side of News, he focuses on positive and transformative advancements in AI, technology, physics, engineering, robotics and space science. Joshua is currently working towards a Bachelor of Science in Business Administration at the University of California, Berkeley. He combines his academic background with a talent for storytelling, making complex scientific discoveries engaging and accessible. His work highlights the innovators behind the ideas, bringing readers closer to the people driving progress.