Scientists make first-ever observation of ‘negative time’
Scientists reveal quantum mechanics’ puzzling “negative time” phenomenon, challenging traditional views of light and time in groundbreaking experiments.
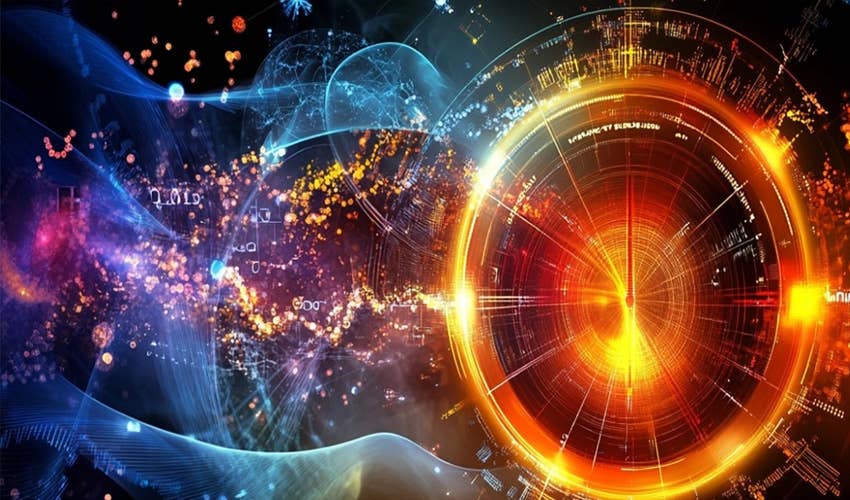
Researchers at the University of Toronto demonstrate the existence of “negative time”. (CREDIT: CC BY-SA 4.0)
The interaction between light and matter has intrigued scientists for centuries. Central to this exploration is the behavior of photons—particles of light—when they travel through various media.
This journey involves complex interactions, including absorption and re-emission by atoms, that temporarily put the atoms into higher-energy states before they return to normal. These phenomena underpin groundbreaking technologies, such as quantum memories, and have opened new frontiers in nonlinear optics.
In a landmark experiment, researchers at the University of Toronto have delved into the puzzling concept of "negative time." Their findings, though not yet peer-reviewed, challenge long-held assumptions about time and energy in quantum mechanics.
Professor Aephraim Steinberg, who led the study, acknowledges the controversy their work has sparked but defends the insights as a crucial step toward understanding the peculiarities of quantum systems.
Quantum Mechanics and “Negative Time”
The notion of “negative time” arises from how photons interact with atoms in a dielectric medium. When light passes through such a material, some photons are absorbed by the atoms and later re-emitted. This interaction creates a temporary “excited” state in the atoms.
Conventional understanding assumed that photons followed a fixed timeline for absorption and re-emission. However, Steinberg’s team demonstrated that these durations can be less than zero, a result they describe as “negative time.”
To illustrate this concept, imagine cars entering a tunnel: if the average entry time for a thousand cars is noon, it might seem odd to observe the first cars exiting slightly earlier, say at 11:59 a.m. While earlier interpretations dismissed such results as artifacts of measurement, the Toronto researchers treated them as significant.
Their work suggests that these counterintuitive timings stem from quantum mechanics—a field known for its probabilistic and non-intuitive nature.
Related Stories
Daniela Angulo, a lead researcher on the team, played a pivotal role in measuring how long atoms remained in their excited states. Using carefully calibrated lasers in a basement laboratory filled with wires and aluminum-wrapped devices, the team optimized their experimental setup over two years.
“That time turned out to be negative,” Steinberg explained. This finding has profound implications for understanding photon behavior in dispersive media.
Negative Time and Group Delay
The group delay, a fundamental concept in light-matter interaction, refers to the time a photon seems to take to traverse a medium. This delay is influenced by the optical depth of the medium and the spectral properties of the light pulse. Steinberg’s team used quantum trajectory theory and weak-value formalism to explore how photons interact with atoms and spend time as atomic excitations.
Their calculations revealed that the time a photon spends as an atomic excitation aligns with the group delay, even when this delay becomes negative. In classical terms, such a delay would be impossible. However, quantum mechanics allows for these anomalous results, which are deeply tied to the probabilistic nature of particle interactions.
This insight was tested experimentally by observing the nonlinear phase shift imprinted on a probe beam, confirming the predictions across a range of optical parameters.
Steinberg likens this phase shift to the π phase-flip that occurs when a broadband pulse travels through an optically dense medium. This phenomenon highlights the intricate interplay between quantum coherence and material properties, challenging traditional assumptions about how light propagates.
The Toronto team’s findings underscore the nuanced behavior of photons and atoms in quantum systems. Their earlier experiments demonstrated that transmitted photons spent nearly as much time in an excited atomic state as scattered photons. These results suggested that a significant fraction of excited atoms contributed to coherent forward emission, a conclusion supported by theoretical models.
In the latest study, the researchers extended these insights, demonstrating that negative group delays are not just mathematical curiosities but observable phenomena. Their experiments also showed that photons carried no information in this process, preserving the integrity of Einstein’s theory of special relativity. This ensures that no physical laws—such as the cosmic speed limit—are violated.
Steinberg emphasizes that the concept of “negative time” does not imply time travel. “We don’t want to say anything traveled backward in time,” he clarified. Instead, these results illuminate the complex and sometimes counterintuitive dynamics of quantum systems, where particles do not adhere to fixed timelines but operate within a spectrum of possible behaviors.
Broader Implications and Skepticism
While the findings have attracted global attention, they have also faced skepticism. German theoretical physicist Sabine Hossenfelder criticized the interpretation of “negative time” in a widely viewed YouTube video. She argued that this term misrepresents what the experiments reveal about photon behavior and phase shifts in a medium.
“The negative time in this experiment has nothing to do with the passage of time,” Hossenfelder stated. “It’s just a way to describe how photons travel through a medium and how their phases shift.”
Angulo and Steinberg, however, maintain that their work addresses critical gaps in understanding light’s interaction with matter. They argue that negative group delays provide new insights into the behavior of light in dispersive media, which could have far-reaching implications for quantum optics and photonic technologies.
The researchers also defended their choice of terminology, acknowledging that it provokes debate but also stimulates deeper discussions about the nature of quantum phenomena. “We’ve made our choice about what we think is a fruitful way to describe the results,” Steinberg said. He noted that while practical applications remain speculative, their findings lay the groundwork for exploring new aspects of quantum physics.
As the debate over “negative time” unfolds, the Toronto team’s work exemplifies the spirit of scientific inquiry. By challenging conventional wisdom and pushing the boundaries of what is measurable, they invite the scientific community to reconsider long-held assumptions about time, light, and quantum mechanics.
Their research, though still in its early stages, opens new avenues for studying light-matter interactions and the role of group delays in quantum systems. Whether or not “negative time” becomes an accepted term, the insights it represents will likely influence the trajectory of quantum physics for years to come.
Note: Materials provided above by The Brighter Side of News. Content may be edited for style and length.
Like these kind of feel good stories? Get The Brighter Side of News' newsletter.
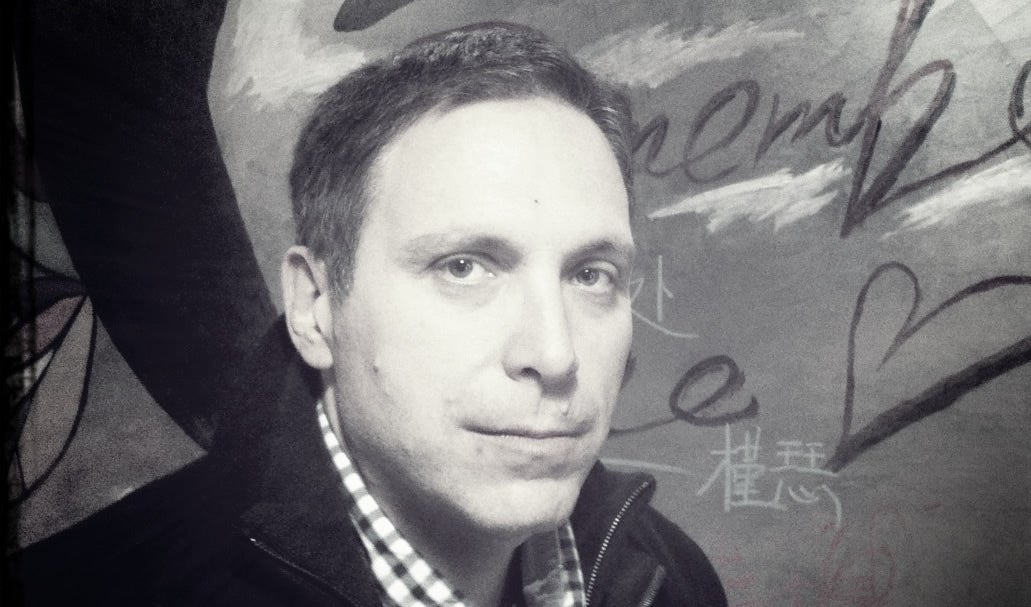