Scientists just made the impossible possible by bending atoms
High-energy helium and hydrogen atoms diffracted through graphene mark a quantum milestone, paving the way for groundbreaking technologies in physics.
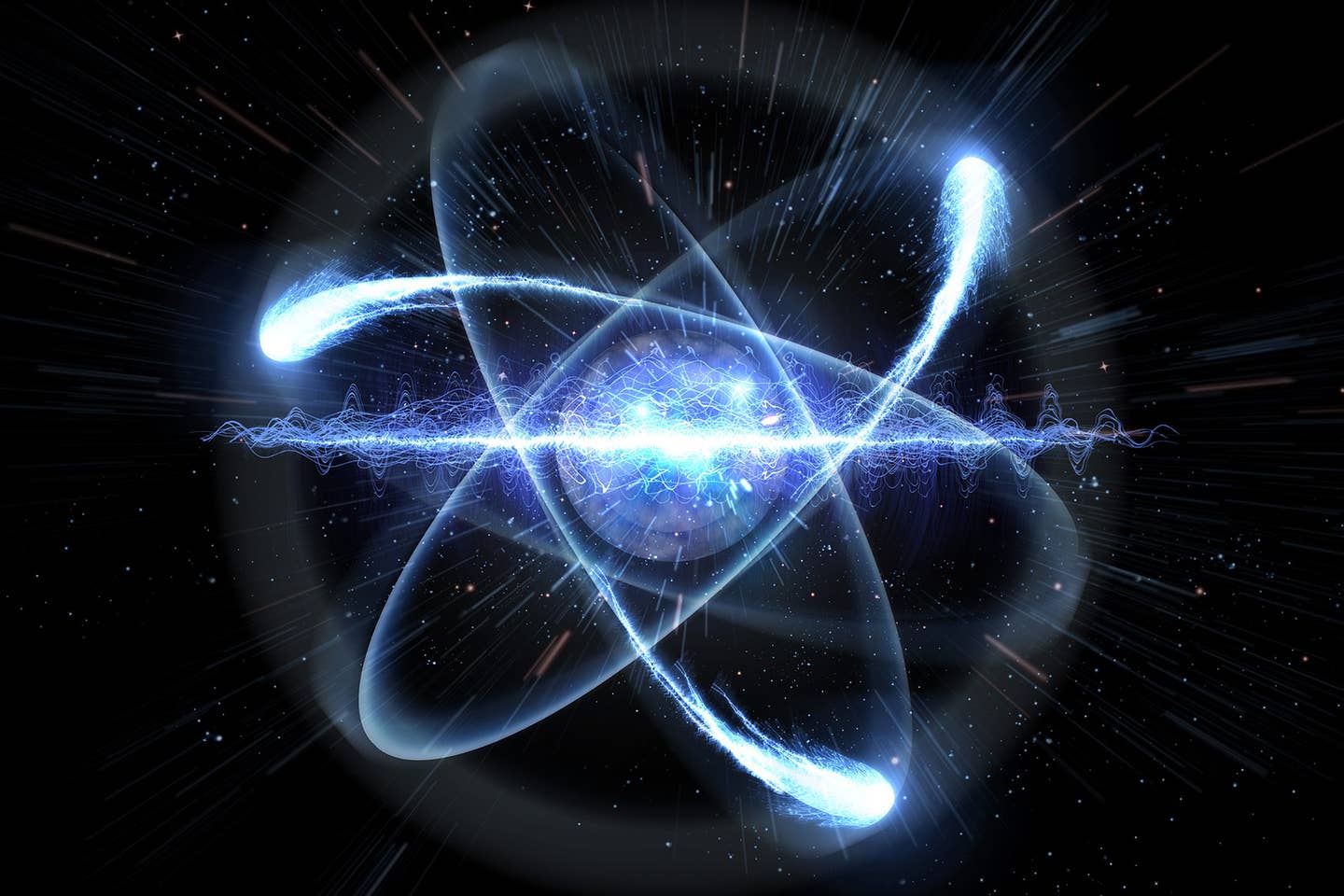
Atomic diffraction through graphene redefines quantum mechanics, unlocking revolutionary technologies like advanced gravitational wave detectors. (CREDIT: CC BY-SA 4.0)
Atomic diffraction has long been a cornerstone of quantum research, offering insights into the wave-like nature of particles.
Recent advancements in this field have pushed the boundaries of what was once thought possible, demonstrating diffraction of high-energy helium and hydrogen atoms through single-layer graphene. This breakthrough not only challenges long-held assumptions but also opens new doors to technologies with transformative potential.
The foundation of particle diffraction experiments traces back to 1927, when George Paget Thomson revealed that electrons passing through a crystal grating produced diffraction patterns, hallmark evidence of their wave-like behavior.
This discovery earned Thomson a Nobel Prize and catalyzed innovations such as electron microscopy. Over the decades, the quest to replicate this effect with atoms, instead of electrons, presented formidable challenges. While electrons could pass through crystalline materials without significant interaction, atoms, being larger and more complex, were expected to damage any crystalline grating they encountered.
Despite advancements in quantum science, atomic diffraction remained elusive. Conventional diffraction methods relied on engineered gratings with relatively large gaps, limiting the sensitivity and precision of experiments. The need for smaller grating periods and higher momentum transfer led researchers to crystalline materials.
However, achieving atomic diffraction through crystals required overcoming the apparent incompatibility between the high energy of atoms and the fragile nature of crystal lattices.
Breakthrough With Graphene Gratings
The turning point came with the use of graphene—a single layer of carbon atoms known for its remarkable strength and stability. Scientists directed helium and hydrogen atoms, accelerated to energies between 400 and 1600 electron volts (eV), at a graphene sheet. Unlike earlier predictions, the high-energy atoms neither damaged the graphene nor disrupted their wave coherence.
Related Stories
Bill Allison of the University of Cambridge likens this to "silently opening and closing a door in a crowded room." The interaction between the atoms and graphene was so subtle that it left no detectable trace, allowing the atoms to maintain their wave-like properties.
The result was the first successful demonstration of atomic diffraction through a crystalline material, producing clear circular diffraction patterns. The key to this success lay in the energy dynamics. By precisely controlling the kinetic energy of the atoms, researchers minimized momentum transfer and energy loss to the graphene lattice.
For helium, a beam energy between 400 and 600 eV offered an optimal balance, preserving coherence while mitigating energy loss. Atomic hydrogen, though lighter, required even more careful tuning due to its stronger coupling with graphene's electronic structure.
Implications for Quantum Research
This experiment represents a monumental leap in quantum mechanics, as it extends atomic diffraction into a previously uncharted energy regime. It confirms that even under conditions of significant energy exchange, the coherence of matter waves can be preserved. Such findings redefine the limits of atomic diffraction, unlocking new possibilities for fundamental and applied quantum research.
Atom interferometry, a field reliant on the sensitivity of matter waves to external forces, stands to benefit immensely. Traditional interferometers, using optical lattices and laser-based gratings, have achieved impressive momentum transfers.
However, graphene’s atomic-scale lattice spacing surpasses these techniques, enabling far greater precision. This advancement could lead to the development of quantum sensors with unprecedented sensitivity, capable of detecting minute variations in gravitational fields or probing fundamental constants with enhanced accuracy.
Moreover, the experiment has implications for understanding decoherence—the process by which quantum systems lose their wave-like properties due to interactions with the environment. By studying the interaction between high-energy atoms and graphene, researchers can explore decoherence mechanisms in a controlled setting, potentially informing the development of more robust quantum technologies.
Revolutionary Applications
The potential applications of this discovery extend beyond the laboratory. One promising avenue is the creation of advanced gravitational wave detectors. Current technologies rely on laser-based interferometers, such as those used in the groundbreaking LIGO and Virgo experiments. However, atomic interferometers leveraging graphene diffraction could achieve even greater sensitivity, enabling the detection of gravitational waves from previously undetectable sources.
Additionally, the precision of atomic diffraction could revolutionize navigation and geophysics. Quantum sensors based on matter waves can measure variations in gravitational fields with extreme accuracy, offering applications in resource exploration, environmental monitoring, and even space exploration.
The ability to manipulate and study atomic matter waves at such a fine scale also paves the way for advancements in quantum computing and communication, where coherence and precise control of quantum states are paramount.
This achievement underscores the ever-evolving nature of scientific discovery. By revisiting the principles laid out nearly a century ago and applying modern techniques, researchers have overcome what was once considered an insurmountable challenge. The use of graphene as a grating material not only highlights the material's versatility but also exemplifies the power of interdisciplinary approaches in science.
As scientists continue to explore the possibilities of atomic diffraction, the potential for groundbreaking innovations remains vast.
This experiment serves as a reminder that the frontiers of science are not static but constantly expanding, driven by curiosity, ingenuity, and the relentless pursuit of knowledge.
Note: Materials provided above by The Brighter Side of News. Content may be edited for style and length.
Like these kind of feel good stories? Get The Brighter Side of News' newsletter.
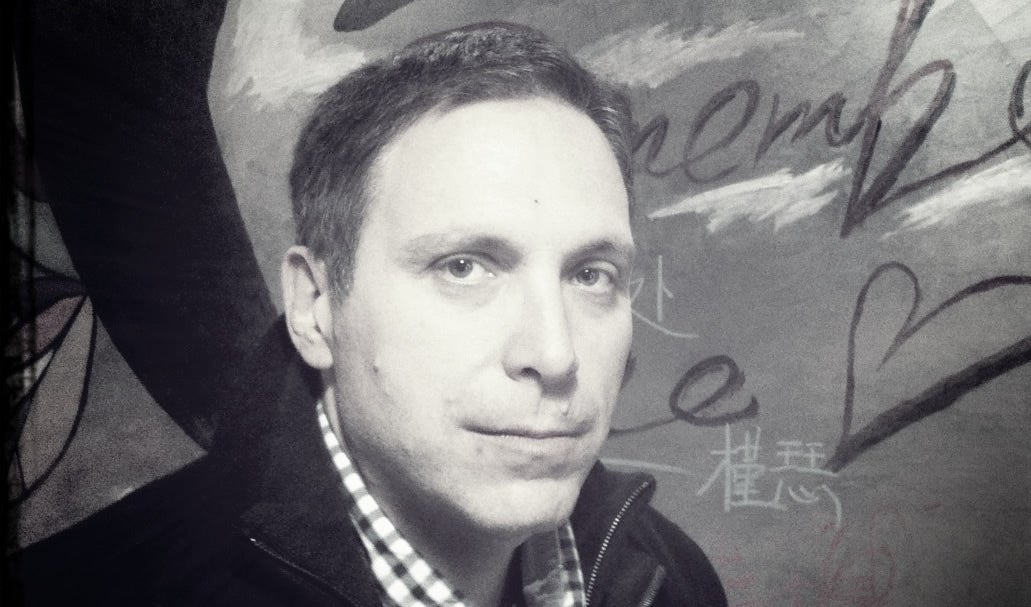
Joseph Shavit
Head Science News Writer | Communicating Innovation & Discovery
Based in Los Angeles, Joseph Shavit is an accomplished science journalist, head science news writer and co-founder at The Brighter Side of News, where he translates cutting-edge discoveries into compelling stories for a broad audience. With a strong background spanning science, business, product management, media leadership, and entrepreneurship, Joseph brings a unique perspective to science communication. His expertise allows him to uncover the intersection of technological advancements and market potential, shedding light on how groundbreaking research evolves into transformative products and industries.