Scientists just discovered a new kind of magnetism
Magnetism, the phenomenon that allows objects to attract or repel one another, is commonly associated with materials like iron and nickel
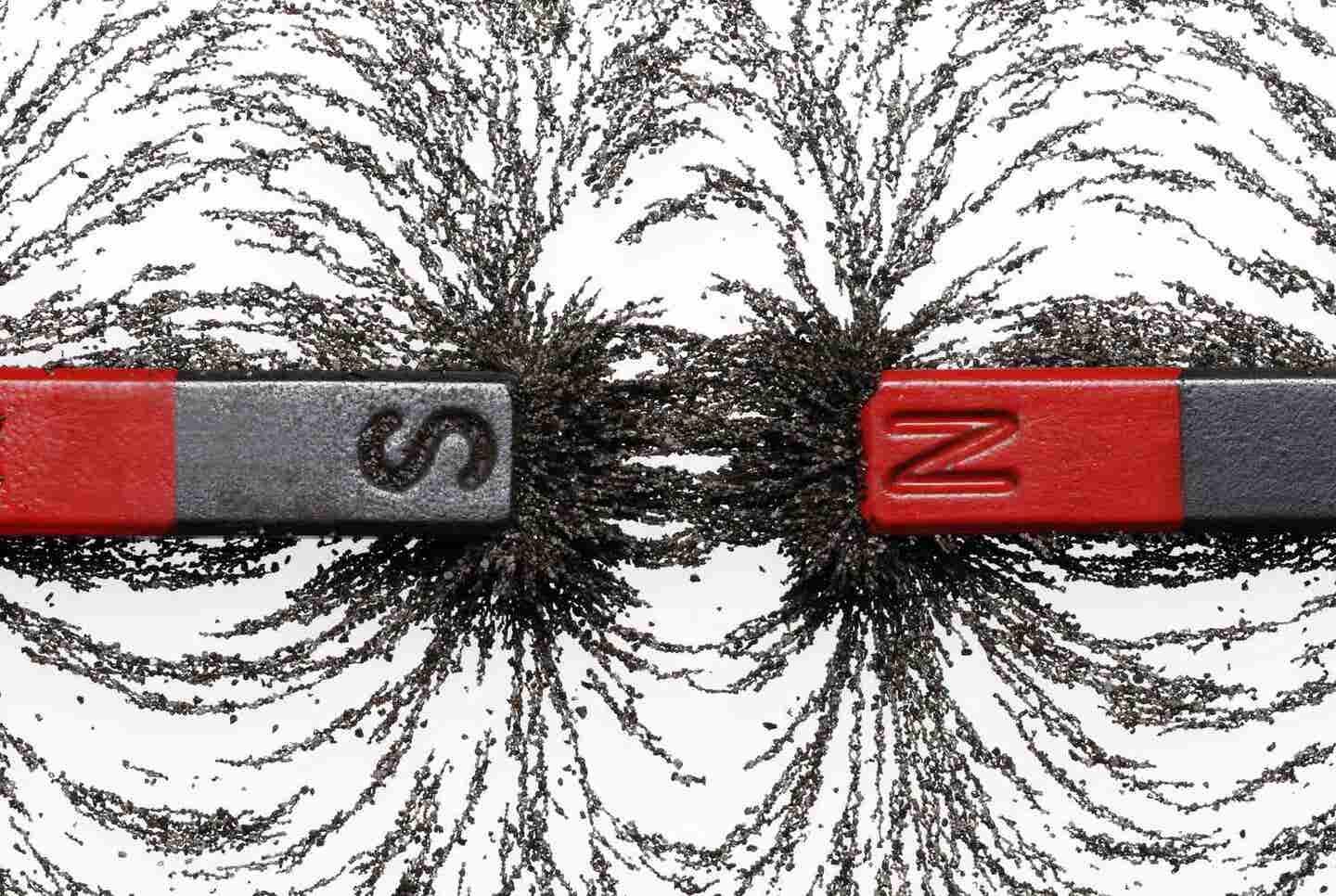
[Dec. 15, 2022: JD Shavit, The Brighter Side of News]
The humble magnet adhering to your refrigerator door may seem like an everyday object, but beneath its surface lies a complex interplay of physical forces. (CREDIT: Getty Images)
In the realm of science, even the simplest phenomena often harbor intricate secrets waiting to be unraveled. The humble magnet adhering to your refrigerator door may seem like an everyday object, but beneath its surface lies a complex interplay of physical forces. Understanding what makes magnets magnetic has long been a fascination for physicists, and recent research at ETH Zurich has uncovered a new chapter in this magnetic saga.
Magnetism, the phenomenon that allows objects to attract or repel one another, is commonly associated with materials like iron and nickel, known as ferromagnetic substances. These materials exhibit magnetic properties as long as they remain below a certain critical temperature. The source of this phenomenon is attributed to the alignment of the magnetic moments of electrons within the material.
Surprisingly, these magnetic moments tend to align in a single direction, even in the absence of an external magnetic field, thanks to a phenomenon known as the exchange interaction. The exchange interaction arises from a combination of electrostatic repulsion between electrons and the quantum mechanical behavior of electron spins, which, in turn, give rise to magnetic moments.
However, researchers at ETH Zurich, led by Ataç Imamoğlu at the Institute for Quantum Electronics and Eugene Demler at the Institute for Theoretical Physics, have recently shattered conventional wisdom by discovering a new form of ferromagnetism in an artificially engineered material.
Related Stories
This novel magnetism emerges through an entirely different mechanism than the well-established exchange interaction. Their groundbreaking findings have been published in the prestigious scientific journal Nature.
Creating an artificial material with unique properties was the first step in unraveling this magnetic enigma. In their laboratory, Imamoğlu's team, including PhD student Livio Ciorciaro and post-doc Tomasz Smolenski, engineered a special material by stacking atomically thin layers of two distinct semiconductor materials, molybdenum diselenide and tungsten disulfide.
The key to their discovery lies in the interaction between these materials, specifically the differences in their lattice constants, which determine the separation between their atoms. The result of this interaction is a two-dimensional periodic potential with a notably large lattice constant, approximately thirty times larger than that of the individual semiconductors. By applying an electric voltage, this unique "moire" material can be filled with electrons, opening the door to exciting investigations into the quantum effects of strongly interacting electrons.
Low temperature data, Sample I. Degree of polarization of the AP resonance as function of magentic field and linear fit around Bz = 0 yielding the susceptibility. (CREDIT: ResearchGate)
Ataç Imamoğlu explains the significance of their work, stating, "Such moiré materials have attracted great interest in recent years, as they can be used to investigate quantum effects of strongly interacting electrons very well. However, so far very little was known about their magnetic properties."
To delve into the magnetic properties of this moiré material, Imamoğlu and his team embarked on a quest to determine whether, for a specific electron filling, the material exhibited paramagnetism, characterized by randomly oriented magnetic moments, or ferromagnetism. They employed a laser-based technique, illuminating the material and measuring the intensity of reflected light for different polarizations.
Illustration of hopping processes for holes and doublons. The presence of long-range interactions introduces an assisted hopping A that modifies the doublon hopping strength. (CREDIT: ResearchGate)
The polarization of light indicates the direction in which its electromagnetic field oscillates, and the material's magnetic moments - driven by the orientation of electron spins - dictate how strongly it reflects a particular polarization. This disparity in reflection helps in deducing whether the spins are aligned in the same direction or different directions, thereby revealing the material's magnetization.
The researchers carefully increased the voltage applied to the material, incrementally filling it with electrons and monitoring the resulting magnetization. Interestingly, up to a specific electron filling - precisely one electron per site of the moiré lattice, known as a Mott insulator - the material exhibited paramagnetic behavior. However, as more electrons were added to the lattice, an unexpected transformation occurred. The material abruptly displayed characteristics akin to ferromagnetism.
Moir´e potential in the conduction band. Electrons occupy the potential minima at the MM sites. (CREDIT: ResearchGate)
Imamoğlu elaborates on this intriguing discovery, stating, "That was striking evidence for a new type of magnetism that cannot be explained by the exchange interaction." If the exchange interaction were solely responsible for this magnetism, its effects would have manifested even with fewer electrons in the lattice. The sudden onset of ferromagnetism hinted at an entirely different underlying mechanism.
Eugene Demler, working in collaboration with post-doc Ivan Morera, provided the missing piece of the puzzle. They proposed that the observed phenomenon could be linked to a mechanism initially theorized by Japanese physicist Yosuke Nagaoka in 1966. In this mechanism, electrons align their spins in the same direction to minimize their kinetic energy (the energy associated with motion), which is significantly larger than the exchange energy.
High temperature data, Sample II. Inverse slope dρAP/dBmeasured at ν= 0.75 as function of temperature for different excitation powers. (CREDIT: ResearchGate)
In the ETH Zurich experiment, this alignment occurs as soon as there is more than one electron per lattice site within the moiré material. Consequently, pairs of electrons, referred to as doublons, team up and spread out across the entire lattice through quantum mechanical tunneling to minimize kinetic energy. However, this phenomenon can only occur if the individual electrons within the lattice align their spins in a ferromagnetic manner. Otherwise, quantum mechanical superposition effects that facilitate the free expansion of doublons would be disrupted.
Imamoğlu underscores the significance of their findings, noting, "Up to now, such mechanisms for kinetic magnetism have only been detected in model systems, for example in four coupled quantum dots, but never in extended solid-state systems like the one we use."
In the moiré material produced at ETH, the electron spins are disordered if there is exactly one electron per lattice site (left). As soon as there are more electrons than lattice sites (right) and pairs of electrons can form doublons (red), the spins align ferromagnetically as this minimizes the kinetic energy. (CREDIT: ETH Zurich)
Looking ahead, the researchers plan to further explore this unconventional ferromagnetism by manipulating the parameters of the moiré lattice. They aim to investigate whether this unique magnetic behavior persists at higher temperatures, as in the current experiment, the material had to be cooled to a mere fraction of a degree above absolute zero to manifest these magnetic properties.
The discovery of this novel form of ferromagnetism in an artificially created moiré material has opened a new frontier in the field of condensed matter physics. The unexpected mechanism underlying this magnetism challenges established theories and paves the way for future exploration of kinetic magnetism in solid-state systems.
As scientists continue to delve into the mysteries of magnetism, their discoveries may not only expand our fundamental understanding of the universe but also lead to groundbreaking technological advancements in the future.
Note: Materials provided above by The Brighter Side of News. Content may be edited for style and length.
Like these kind of feel good stories? Get the Brighter Side of News' newsletter.
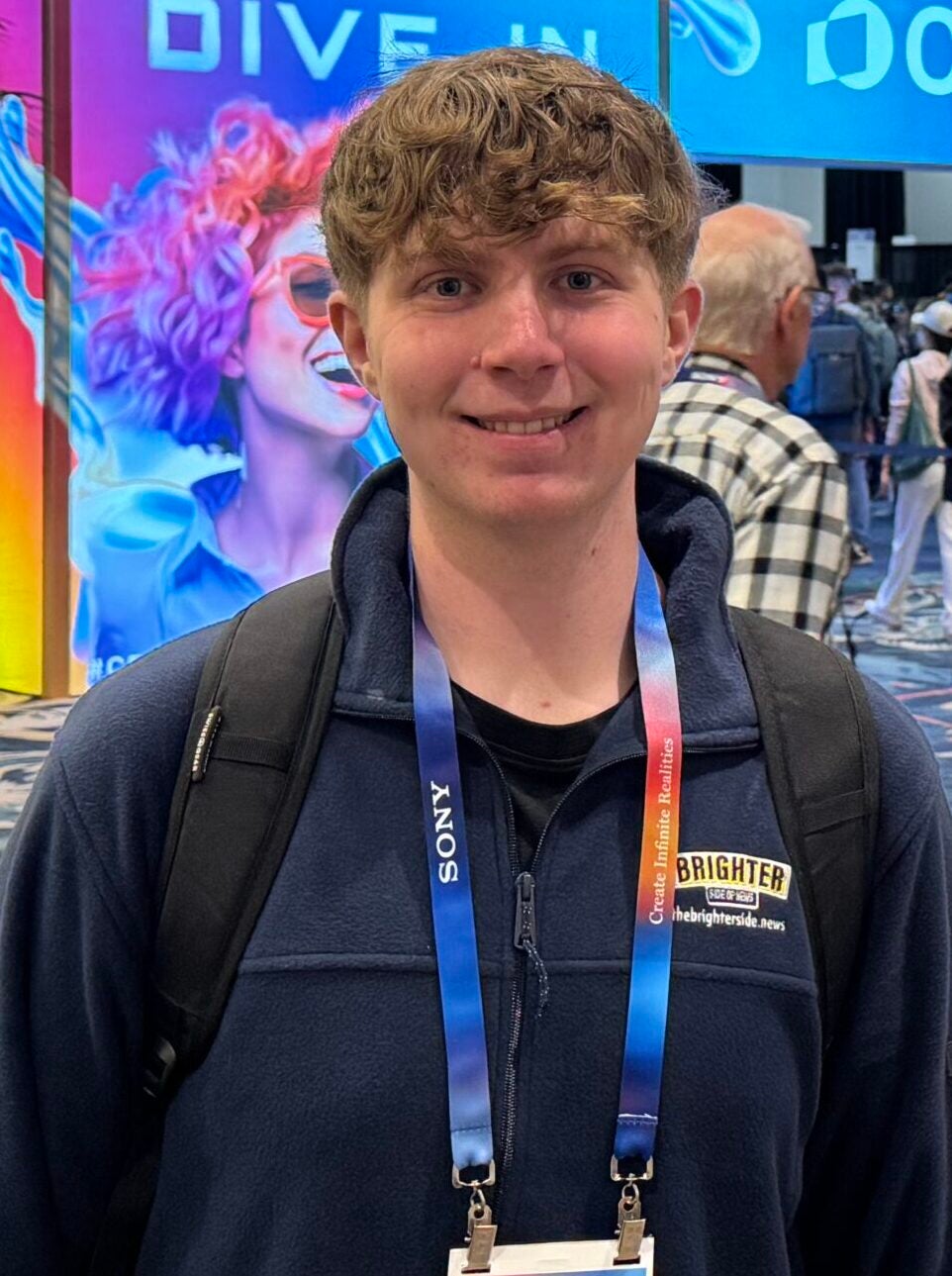
Joshua Shavit
Science & Technology Writer | AI and Robotics Reporter
Joshua Shavit is a Los Angeles-based science and technology writer with a passion for exploring the breakthroughs shaping the future. As a contributor to The Brighter Side of News, he focuses on positive and transformative advancements in AI, technology, physics, engineering, robotics and space science. Joshua is currently working towards a Bachelor of Science in Business Administration at the University of California, Berkeley. He combines his academic background with a talent for storytelling, making complex scientific discoveries engaging and accessible. His work highlights the innovators behind the ideas, bringing readers closer to the people driving progress.