Scientists finally understand how animals get their stripes and spots
Nature has always been a canvas of intricate designs, from the majestic spots adorning leopards to the mesmerizing stripes that adorn zebras
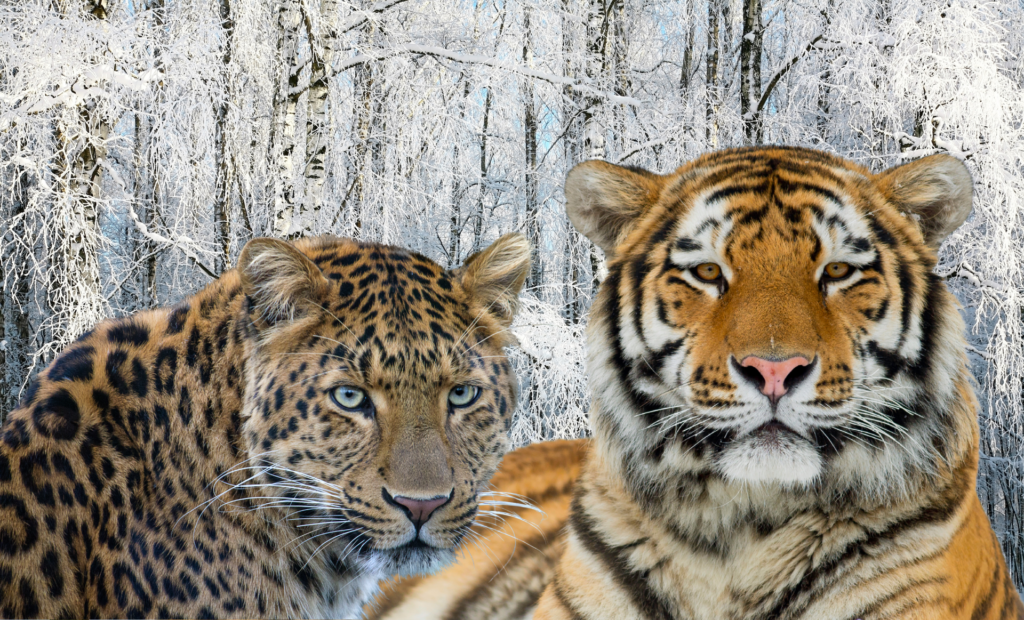
[Dec. 22, 2023: JD Shavit, The Brighter Side of News]
Nature has always been a canvas of intricate designs, from the majestic spots adorning leopards to the mesmerizing stripes that adorn zebras. (CREDIT: Creative Commons)
Nature has always been a canvas of intricate designs, from the majestic spots adorning leopards to the mesmerizing stripes that adorn zebras, and even the precise hexagons embellishing the skin of boxfish.
The formation of these patterns has long mystified scientists, but a groundbreaking study by engineers at the University of Colorado Boulder suggests an unexpected connection between the process of removing dirt from laundry and the development of vibrant patterns in tropical fish. Published in the prestigious journal Science Advances, their research may provide answers to age-old questions about the origins of biological patterns.
Benjamin Alessio, the paper's lead author and an undergraduate researcher in the Department of Chemical and Biological Engineering at CU Boulder, pointed out that the fundamental question of pattern development in biology revolves around how complex shapes and designs emerge from the initially simple configuration of a clump of cells. Alessio stated, "Our work uses a simple physical and chemical mechanism to explain a complicated biological phenomenon."
While genes undoubtedly play a pivotal role in encoding information for patterns, such as the color of a leopard's spots, they do not provide a complete understanding of the precise locations where these patterns will form.
Related Stories
In 1952, well before the discovery of DNA's double helix structure, Alan Turing, the father of modern computing, proposed a daring theory to explain the emergence of animal patterns.
Turing's hypothesis revolved around the idea that as tissues develop, they release chemical agents that diffuse through the tissue, much like how milk disperses in coffee. These agents would either react with each other to form spots or inhibit the spread and reaction of the agents, creating spaces between the spots. Turing's theory challenged the notion that complex genetic processes were solely responsible for biological pattern formation, suggesting that a simple reaction-diffusion model might suffice.
However, as Ankur Gupta, the corresponding author and an assistant professor in the Department of Chemical and Biological Engineering, pointed out, Turing's mechanism could produce patterns, but they lacked the sharpness and precision seen in nature. For instance, when milk diffuses in coffee, it creates a blurry and ill-defined pattern. This lack of clarity in Turing's model left a significant gap in explaining the intricate patterns observed in animals.
Top: A male Ornate Boxfish (Aracana ornata). Bottom left: A close-up picture of the fish’s natural hexagonal pattern. Bottom center: Fish pattern simulation based on Turing’s reaction-diffusion theory. Bottom right: Diffusiophoresis-enhanced reaction-diffusion simulation. (CREDIT: The Birch Aquarium/Scripps Institution of Oceanography and Benjamin Alessio)
Alessio's inspiration for bridging this gap came during a visit to the Birch Aquarium in San Diego, where he was captivated by the remarkable sharpness of the hexagonal pattern on a boxfish's skin. This intricate pattern consisted of vibrant purple dots enclosed within well-defined hexagonal outlines, separated by thick black spacing. It was reminiscent of the sharp patterns he had observed in his computer simulations. Intrigued, Alessio wondered if a phenomenon known as diffusiophoresis could shed light on the formation of such crisp patterns in nature.
Diffusiophoresis occurs when molecules move through a liquid medium in response to concentration differences, enhancing the movement of other molecules within the same environment. This seemingly obscure concept plays a pivotal role in everyday life, particularly in the process of laundering clothes.
Top: A Jeweled Moray Eel (Muraena lentiginosa). Bottom left: A close-up picture of the eel's natural pattern. Bottom center: Pattern simulation based on Turing’s reaction-diffusion theory. Bottom right: Diffusiophoresis-enhanced reaction-diffusion simulation. (CREDIT: craigjhowe/iNaturalist and Benjamin Alessio)
Recent research has demonstrated that rinsing soap-soaked clothes in clean water effectively removes dirt faster than rinsing them in soapy water. The reason lies in the diffusion of soap molecules from the fabric into water with a lower soap concentration. As soap molecules migrate into the water, they draw dirt out of the fabric. In contrast, when clothes are immersed in soapy water, where there is no significant difference in soap concentration, the dirt remains trapped.
What sets diffusiophoresis apart is the predictability of molecular movement it entails. Gupta and Alessio observed in their simulations that molecules following the principles of diffusiophoresis consistently follow clear trajectories, resulting in patterns with sharp, well-defined outlines.
Diffusiophoretic enhancement for various reaction-diffusion mechanisms. (CREDIT: Science Advances)
To investigate whether diffusiophoresis could play a role in shaping animal patterns, Gupta and Alessio embarked on a simulation of the ornate boxfish's purple and black hexagonal pattern, initially using only Turing's equations. The outcome was a picture of blurred purple dots surrounded by faint black outlines. However, when they modified the equations to incorporate diffusiophoresis, a remarkable transformation occurred. The simulated pattern closely resembled the vivid and sharp bi-color hexagonal design found on the boxfish.
Their groundbreaking theory suggests that as chemical agents diffuse through tissues, as Turing proposed, they also induce the movement of pigment-producing cells through diffusiophoresis. This dynamic process is akin to how soap molecules extract dirt from laundry. The pigment cells are then arranged in patterns with far sharper outlines than previously envisioned.
Decades after Turing introduced his pioneering theory, scientists have employed this mechanism to explain various patterns in biology, such as the distribution of hair follicles in mice and the formation of ridges in the palates of mammals. This profound insight into pattern formation has far-reaching implications beyond biology.
Gupta expressed his hope that their study, along with ongoing research within his group, will not only enhance our understanding of pattern formation but also inspire scientists to develop innovative materials and potentially life-changing medicines. He said, "Our findings emphasize that diffusiophoresis may have been underappreciated in the field of pattern formation. This work not only has the potential for applications in the fields of engineering and materials science but also opens up the opportunity to investigate the role of diffusiophoresis in biological processes, such as embryo formation and tumor formation."
By incorporating diffusiophoresis into Turing's classic reaction-diffusion model, engineers have provided a compelling explanation for the sharp and well-defined patterns observed in nature. Nature's patterns, it seems, have a lot more in common with your laundry than you might have ever imagined.
Note: Materials provided above by the The Brighter Side of News. Content may be edited for style and length.
Like these kind of feel good stories? Get the Brighter Side of News' newsletter.
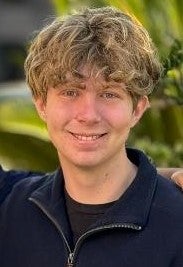