Scientists finally solve thirty-year-old black hole mystery
Resonant interactions between black hole quasinormal modes have unlocked a 30-year-old gravitational wave mystery.
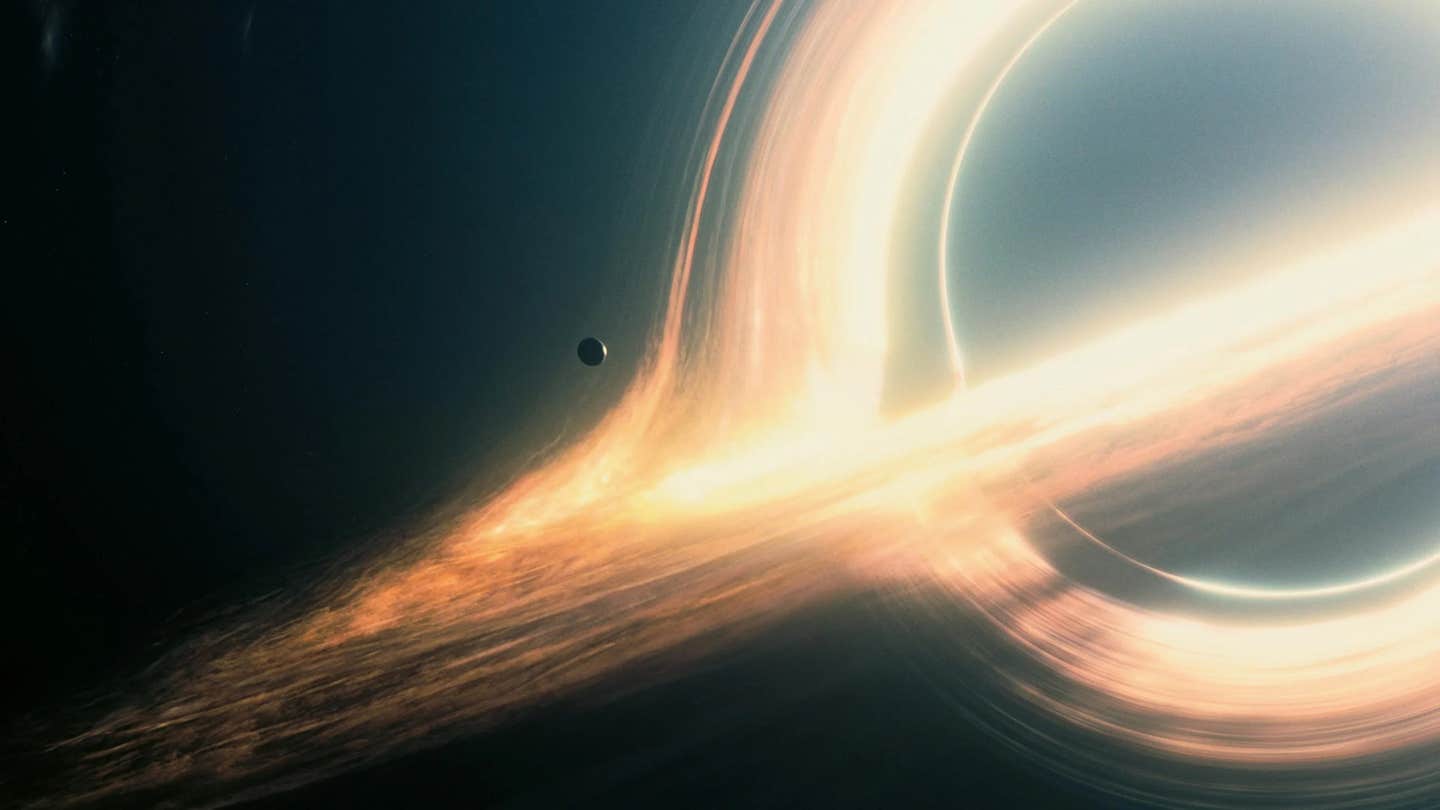
General relativity predicts that the only rotating black hole solution is the Kerr spacetime. (CREDIT: CC BY-SA 4.0)
The last decade has brought a new way to explore the universe. Gravitational wave astronomy, made possible by observatories like LIGO, Virgo, and KAGRA, now lets scientists study the universe through ripples in spacetime. These efforts have helped researchers understand the behavior of black holes, the densest objects in the universe where even light cannot escape.
Black holes are simpler than you might think. General relativity predicts that the only rotating black hole solution is the Kerr spacetime. This means a black hole can be fully described by just two properties: mass and spin. After two black holes collide, the merged black hole settles into this Kerr state, sending out gravitational waves during the process known as the "ringdown."
Ringdown Waves and Quasinormal Modes
The gravitational waves from the ringdown are made up of special signals called quasinormal modes, or QNMs. Each QNM looks like a vibration that fades over time, much like the way a struck bell slowly goes silent. These modes are defined by complex frequencies and excitation factors, both determined solely by the black hole's mass and spin.
For years, researchers have studied how these QNMs behave when a black hole's parameters change. While a lot of progress has been made, some strange behaviors in the QNM patterns remained unexplained. Among them was an odd "dissonance" first spotted by Hisashi Onozawa at Tokyo Institute of Technology back in 1997.
Onozawa’s calculations revealed that one specific QNM did not behave smoothly like the others. Scientists suspected a mistake, but even with improved computers and methods, the mystery remained.
The Resonance Phenomenon Near Exceptional Points
A breakthrough finally came from Associate Professor Hayato Motohashi at Tokyo Metropolitan University. Using new high-precision computing and the ideas from non-Hermitian physics, Motohashi discovered that the dissonance wasn’t a simple glitch. It was a true physical phenomenon, caused by the resonant interaction of two different QNMs.
Related Stories
This phenomenon is called "resonant excitation" and is linked to something known in quantum mechanics as an avoided crossing. Usually, two energy levels in a system can’t overlap unless a special condition is met. Near an exceptional point—a special type of singularity in complex systems—these interactions cause dramatic changes. In black holes, these interactions between QNMs cause large shifts in their behavior.
Motohashi’s work showed that the dissonance observed decades ago was not limited to one mode. In fact, careful studies revealed that many pairs of QNMs exhibit this resonant behavior, especially in high-spin black holes.
Spirals, Loops, and Amplifications
Looking at the (2,2) mode, which is the main gravitational wave signal from a merging black hole, most QNMs shift predictably as spin increases. But at a spin near 90% of the maximum, the fifth overtone suddenly bends and forms a small loop before moving away from the rest.
While previous research focused mainly on this fifth overtone, Motohashi found that the sixth overtone is also slightly affected during this process. Not only do the frequencies shift, but the excitation factors—how strongly a mode is excited—also grow unexpectedly large.
Interestingly, while most modes follow a spiral pattern on the complex plane, the fifth and sixth overtones spiral in opposite directions near this spin value. This showed that the strange behavior was not just about frequency shifts but involved strong amplification as well.
Further study of higher multipole modes, like the (3,1) mode, showed even sharper examples of this phenomenon. For certain pairs of overtones, their frequencies repel each other strongly, forming patterns that resemble classic shapes like hyperbolas and lemniscates—the figure-eight curve—on the complex plane.
The stronger the repulsion between two QNMs, the greater the amplification of their excitation factors. This amplification occurs in a point-symmetric way, another sign that it is a deeply linked phenomenon.
A Universal Phenomenon Across Fields
One key insight from Motohashi's work is that this resonant excitation is not limited to gravitational waves from black holes. Similar behavior shows up in other fields, including optical physics, where scientists study electromagnetic waves. Resonances linked to avoided crossings and exceptional points are common features across many areas of physics.
By plotting the differences between excitation factors and frequencies, Motohashi showed that they follow a precise inverse relationship. In the sharpest cases, the data fit a special type of mathematical curve called a quarter-power Lorentzian very closely. During resonance, the product of the differences remains almost constant, further proving the deep connection between the two.
This new understanding offers fresh hope for black hole spectroscopy. By studying these resonances, scientists might measure black hole properties even more precisely. It could unlock new ways to test general relativity and probe the most extreme environments in the universe.
Motohashi’s findings suggest that the gravitational wave signals detected by LIGO, Virgo, and KAGRA might hold much more information than previously believed. As global-scale experiments become more sensitive, the field of non-Hermitian gravitational physics may lead the way to uncovering hidden structures in spacetime.
By solving a 30-year-old puzzle, Motohashi has opened the door to a new branch of physics. His work shows how ideas from different fields can come together to unlock mysteries that once seemed impossible to solve.
Research findings are available online in the journal Physical Review Letters.
Note: The article above provided above by The Brighter Side of News.
Like these kind of feel good stories? Get The Brighter Side of News' newsletter.
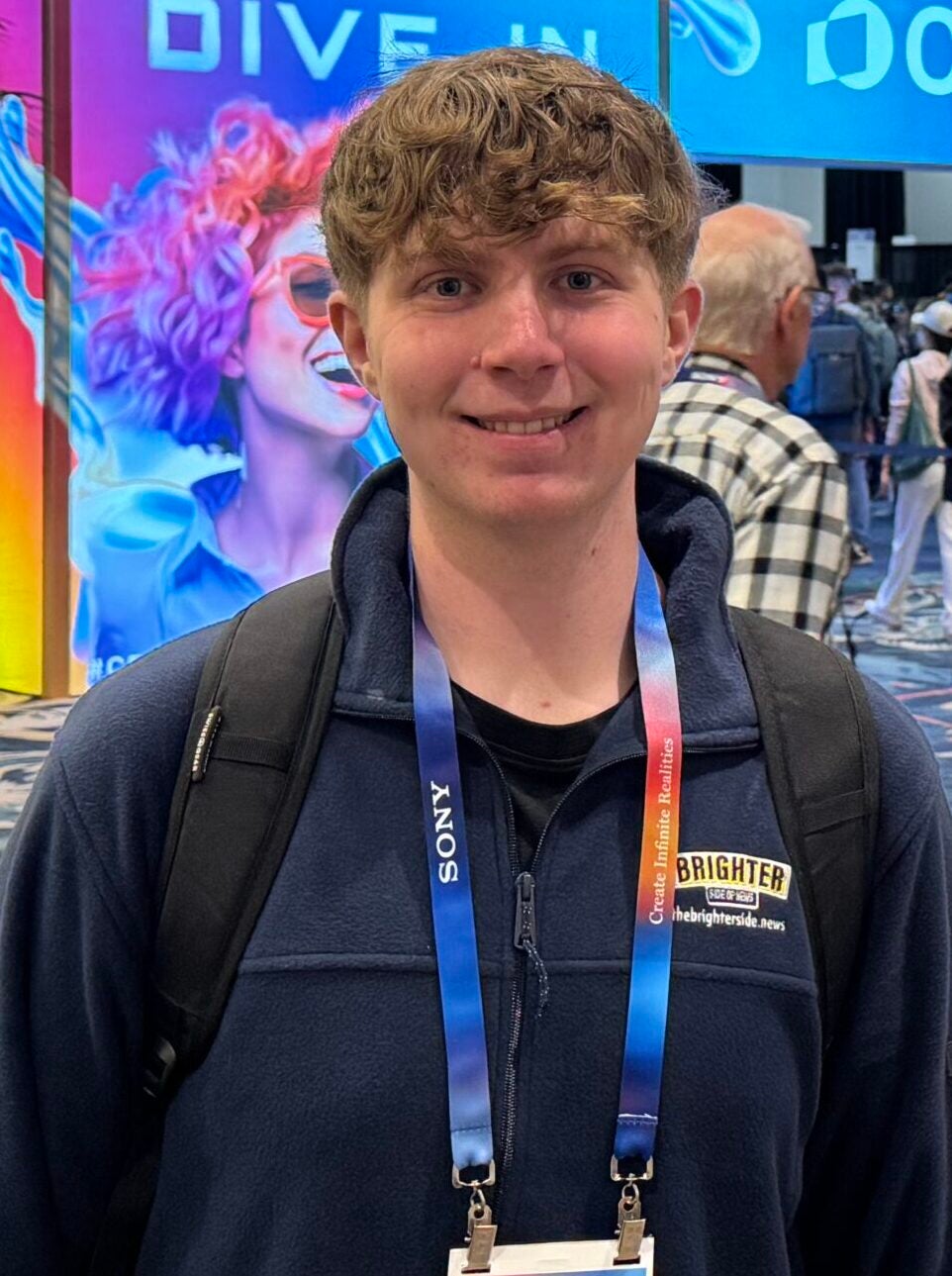
Joshua Shavit
Science & Technology Writer | AI and Robotics Reporter
Joshua Shavit is a Los Angeles-based science and technology writer with a passion for exploring the breakthroughs shaping the future. As a contributor to The Brighter Side of News, he focuses on positive and transformative advancements in AI, technology, physics, engineering, robotics and space science. Joshua is currently working towards a Bachelor of Science in Business Administration at the University of California, Berkeley. He combines his academic background with a talent for storytelling, making complex scientific discoveries engaging and accessible. His work highlights the innovators behind the ideas, bringing readers closer to the people driving progress.