Scientists discover new sources of the molecule that built the universe
New research reveals unexpected sources of H₃⁺ in space, challenging traditional models of interstellar chemistry and star formation.
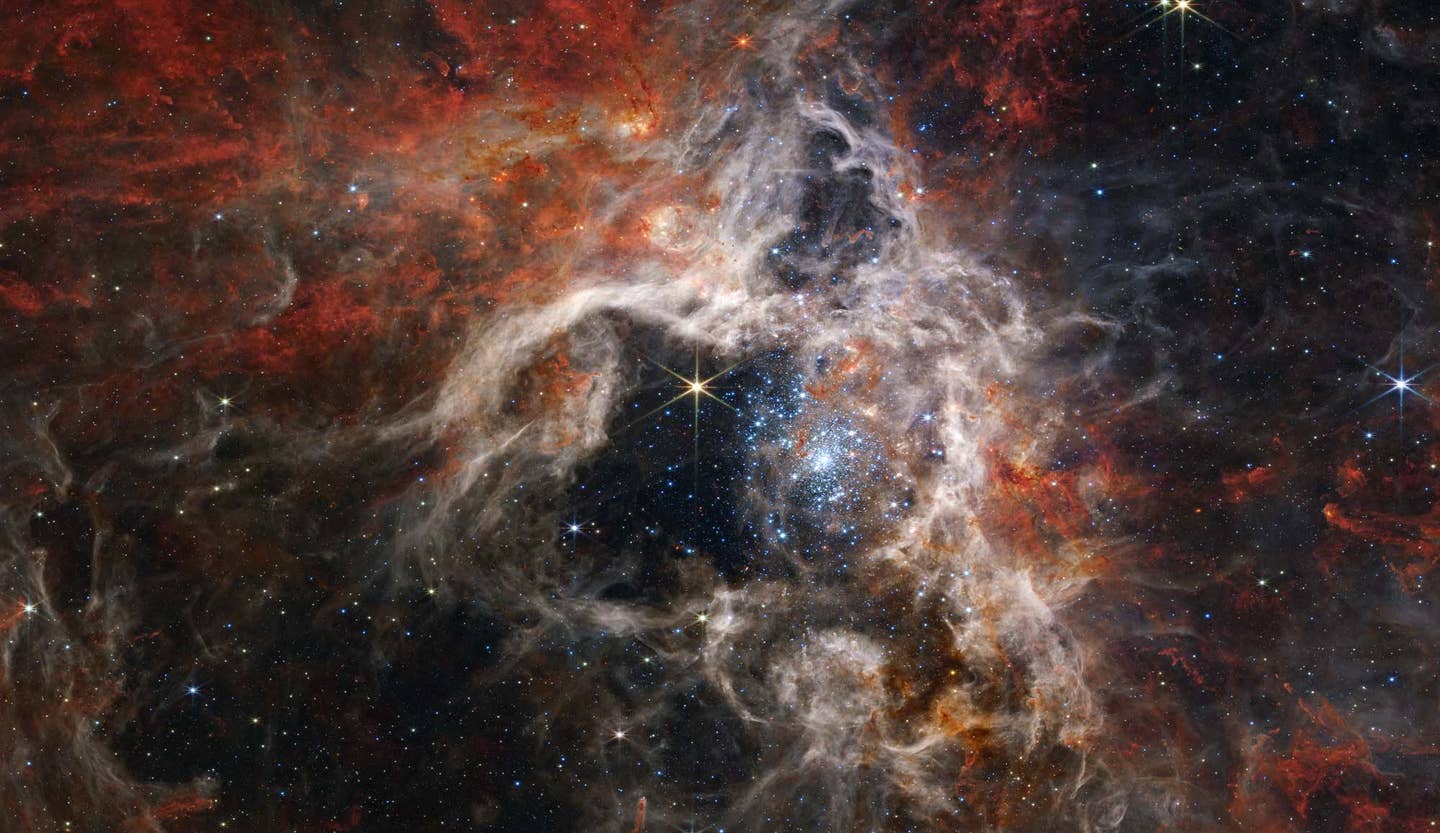
A star-forming region in the Tarantula Nebula as captured by the James Webb Space Telescope. MSU researchers have recently uncovered alternative sources of the molecule H₃⁺, which plays a crucial role in the birth of stars and other cosmic chemistry.
(CREDIT: NASA, ESA, CSA, STScI, Webb ERO Production Team)
In the vast expanse of space, where stars are born and planets take shape, one molecule plays a crucial role in interstellar chemistry.
The trihydrogen cation, H₃⁺, is considered a key player in the formation of new molecules and the cooling of interstellar gas clouds. Its high acidity and ability to transfer protons make it essential in many chemical reactions, including those that set the stage for star formation.
Scientists have long understood the primary way H₃⁺ forms—when a hydrogen molecule (H₂) collides with its ionized counterpart, H₂⁺. However, recent research suggests that alternative pathways also contribute to its abundance in the cosmos.
A new study from Michigan State University (MSU) has uncovered fresh insights into these pathways, particularly in compounds known as methyl halogens and pseudohalogens.
These findings, published in Nature Communications, could reshape the understanding of H₃⁺ formation and its impact on astrochemistry.
A Roaming Mechanism in Doubly Ionized Molecules
Traditionally, the formation of H₃⁺ is thought to occur through the Hogness and Lunn mechanism, in which cosmic rays ionize molecular hydrogen, generating highly reactive species that donate protons to other molecules. However, new evidence points to a more complex and unexpected process.
The MSU team, led by Piotr Piecuch and Marcos Dantus, investigated how H₃⁺ forms in doubly ionized organic molecules. Double ionization occurs when a molecule loses two electrons due to high-energy cosmic rays or laser pulses. In theory, this should cause the molecule to disintegrate immediately due to the repulsion between two positive charges, a phenomenon known as Coulomb explosion. Yet, experiments tell a different story.
Instead of instant fragmentation, a neutral hydrogen molecule (H₂) sometimes remains bound to the doubly ionized molecule. This H₂ molecule then "roams" within the molecular framework, eventually abstracting a proton and forming H₃⁺. The roaming behavior is highly unusual and suggests that H₃⁺ can form in ways previously unconsidered.
Related Stories
Dantus describes the phenomenon: “We demonstrated that the hydrogen didn’t simply fly away, but it stuck around, sometimes for quite a long time. This was highly unusual.”
Methyl Halogens and Pseudohalogens: Unexpected Sources of H₃⁺
To further explore this mechanism, researchers turned to methyl halogens—organic molecules containing a carbon-hydrogen (CH₃) group bonded to a halogen atom, such as chlorine or bromine. Experiments revealed that in some cases, when these molecules undergo double ionization, they follow a similar roaming mechanism to produce H₃⁺.
Methanol (CH₃OH) provides a striking example of this behavior. Studies using femtosecond time-resolved ionization techniques showed that after methanol is doubly ionized, an H₂ molecule forms and moves around within the CHOH₂⁺ dication before abstracting a proton. The process can take as little as 100 femtoseconds—a timescale remarkably close to H₃⁺ formation in interstellar conditions.
A similar pattern appears in methyl chloride (CH₃Cl) and methyl bromide (CH₃Br). The research team found that these compounds readily produce H₃⁺ upon double ionization. However, in methyl fluoride (CH₃F) and methyl iodide (CH₃I), H₃⁺ production is negligible. This trend contradicts expectations based on electronegativity, suggesting other factors influence the reaction.
Even more puzzling is the case of methyl cyanide (CH₃CN), a pseudohalogen-containing compound. Despite having a highly electronegative cyanide group, it barely produces H₃⁺ when doubly ionized. This discrepancy highlights the complexity of the underlying mechanisms and suggests that factors beyond simple charge distribution dictate H₃⁺ formation.
Implications for Astrochemistry and Beyond
Understanding how H₃⁺ forms in different environments is essential for refining models of cosmic chemistry. This molecule is responsible for many fundamental reactions in space, from shaping interstellar clouds to influencing planetary atmospheres. For instance, H₃⁺ is abundant in gas giants like Jupiter and Saturn, where it plays a role in regulating atmospheric chemistry.
With these new insights, scientists can better predict where H₃⁺ forms and in what quantities. As Piecuch explains, “H₃⁺ is a small molecule that might not be as important to us on Earth as water or proteins, but it’s a molecule we truly want to understand in terms of its abundance in the universe, how it is produced, and how fast its chemical reactions are.”
These findings could also impact research beyond astrochemistry. The roaming mechanism may be relevant in other chemical reactions, including those occurring in plasmas, combustion, and even biological systems. By establishing a set of governing factors for H₃⁺ formation, researchers now have a framework to explore its presence in a wide range of molecular environments.
The Future of H₃⁺ Research
While the standard H₂-meets-H₂⁺ pathway remains the primary source of H₃⁺ in the universe, the discovery of alternative formation routes challenges previous assumptions. Molecular clouds contain vast numbers of organic compounds, suggesting that additional, previously unrecognized processes contribute to H₃⁺ production.
Dantus emphasizes this point: “Hydrogen is the most common element in the universe, so H₂ meeting H₂⁺ is still the key. However, there are so many organic molecules in these diffuse molecular clouds that it’s possible a lot of H₃⁺ is still being formed by the processes we’ve studied.”
Even a small increase in H₃⁺ abundance due to these mechanisms could have significant implications. Star formation models, which rely on accurate chemical composition data, may need revision to account for these newly discovered reactions.
Piecuch adds, “Even if there are only a few percent more H₃⁺ molecules in the universe because of the small organic compounds we and others have studied, the models that scientists use to study processes such as star formation may have to be revisited.”
The study’s findings were achieved through a combination of ultrafast laser spectroscopy and high-level quantum chemistry simulations, a collaboration between experimentalists and theorists. The team also created molecular dynamics simulations that visually depict the roaming mechanism, available as supplementary materials in their publication.
By continuing to refine these techniques and explore new molecular candidates, researchers hope to deepen their understanding of H₃⁺ formation. The molecule that helped build the universe still has secrets to reveal.
Note: Materials provided above by The Brighter Side of News. Content may be edited for style and length.
Like these kind of feel good stories? Get The Brighter Side of News' newsletter.