Scientists develop space-age metal that doesn’t expand when heated
Zero thermal expansion material maintains its size across extreme temperatures, offering applications in aerospace, semiconductors, and precision instruments.
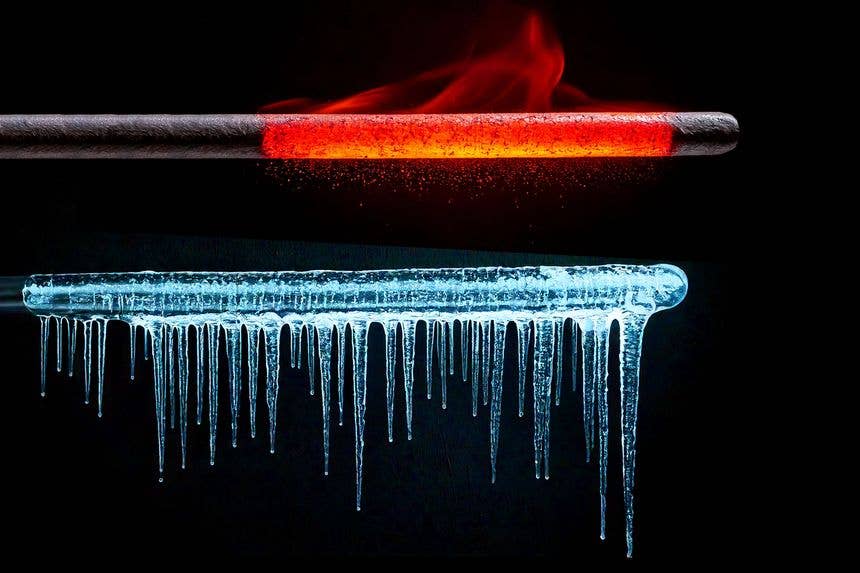
(CREDIT: CC BY-SA 4.0)
The Eiffel Tower grows taller in summer due to thermal expansion. While this effect is common in most metals, it poses challenges in precision engineering. Certain applications demand materials that maintain a stable size regardless of temperature. The search for such materials has led to discoveries in zero thermal expansion (ZTE) materials.
Atoms vibrate more as temperature rises, increasing the average space between them. This leads to expansion in most materials. However, some materials counteract this effect through compensating mechanisms. Magnetic materials, for instance, can contract when their magnetic order weakens with rising temperatures. This balance between expansion and contraction results in a stable material size.
One well-known example is Invar, an iron-nickel alloy with extremely low thermal expansion. However, the underlying physics behind its stability remained unclear. Recent advancements in computer simulations have provided a deeper understanding of this phenomenon, paving the way for new ZTE materials.
A Breakthrough in Zero Thermal Expansion
A collaboration between researchers at TU Wien and the University of Science and Technology Beijing led to the development of a new ZTE material. Using advanced computer models, scientists analyzed atomic-level interactions in magnetic materials. Their simulations revealed how specific electron behaviors influence thermal expansion.
Dr. Sergii Khmelevskyi from TU Wien explains, "The effect is due to certain electrons changing their state as the temperature rises. The magnetic order in the material decreases, causing it to contract. This effect almost exactly cancels out the usual thermal expansion."
With this knowledge, researchers predicted new materials with similar properties. Their predictions were tested in collaboration with an experimental team led by Prof. Xianran Xing and Assoc. Prof. Yili Cao. The result was a new alloy with even better thermal stability than Invar: the pyrochlore magnet. The research findings were published in the journal National Science Review.
Pyrochlore Magnets: A New Class of ZTE Materials
Unlike traditional invar alloys made from two metals, the new pyrochlore magnet consists of four elements: zirconium, niobium, iron, and cobalt. This combination results in an exceptionally low thermal expansion coefficient over a wide temperature range—approximately 3 to 440 K.
Related Stories
The material’s unique behavior stems from its heterogeneous composition. Unlike a perfect crystalline lattice, its structure contains localized variations in element concentration. This non-uniformity allows different regions to respond to temperature changes in complementary ways, achieving near-zero overall expansion.
“The material has an extremely low coefficient of thermal expansion over an unprecedentedly wide temperature range,” says Yili Cao. Its stability makes it highly desirable for precision applications, including aerospace engineering, semiconductor manufacturing, and scientific instruments.
The Role of Magnetic Order in ZTE
Magnetism plays a crucial role in achieving zero thermal expansion. The new pyrochlore magnet is a cubic lattice composed of corner-sharing tetrahedra. This unique arrangement fosters strong interactions between atomic spins, affecting thermal expansion behavior.
Researchers have long studied materials with negative thermal expansion (NTE), where substances shrink as they heat up. These materials, found in oxides, fluorides, and certain metal alloys, have been used to engineer ZTE materials. However, past attempts were limited by narrow temperature ranges and structural impurities.
To enhance ZTE properties, scientists explored different approaches, including element substitution and lattice modifications. In one study, adding germanium altered the distortions in cubic Mn3Cu0.5Ge0.5N, influencing its NTE characteristics. Similarly, controlled doping in ScF3-based materials improved their structural stability.
The new pyrochlore magnet achieves its stability through a nonstoichiometric approach. Excess cobalt atoms alter the lattice’s magnetic interactions, stabilizing the material across a wide temperature range. Experimental techniques like neutron diffraction, Mössbauer spectroscopy, and synchrotron X-ray analysis confirmed these findings.
Beyond thermal stability, this new material exhibits excellent corrosion resistance in both acidic and alkaline environments. This makes it highly promising for real-world applications requiring durability in extreme conditions.
Future Applications of ZTE Materials
ZTE materials have broad applications in industries where thermal stability is critical. In aerospace engineering, components must endure drastic temperature fluctuations without deforming. Satellite systems, for instance, experience temperature swings from -150°C to 150°C in space. A ZTE material prevents structural changes that could affect performance.
In semiconductor manufacturing, even minute expansions can disrupt microscopic circuits. The new pyrochlore magnet could lead to more stable electronic components, enhancing device reliability. High-precision optical instruments also benefit from ZTE materials, ensuring accurate measurements in varying temperatures.
The discovery of this new alloy marks a significant step forward in material science. By understanding atomic-scale interactions, researchers can now design materials with tailored thermal properties. This opens the door to future innovations in engineering and technology.
As research progresses, further refinements may enhance the performance and scalability of ZTE materials. Scientists continue to explore new element combinations and structural modifications to optimize stability. The ability to manipulate atomic interactions offers exciting possibilities for next-generation materials.
The breakthrough in pyrochlore magnets demonstrates how advanced simulations and experimental techniques can transform material science. With practical applications spanning multiple industries, this innovation may soon become a cornerstone of precision engineering.
Note: Materials provided above by The Brighter Side of News. Content may be edited for style and length.
Like these kind of feel good stories? Get The Brighter Side of News' newsletter.