Science breakthrough: Researchers make electrons visible in slow motion
Understanding how the electron collective movement is halted allows for the targeted development of materials with specific properties.
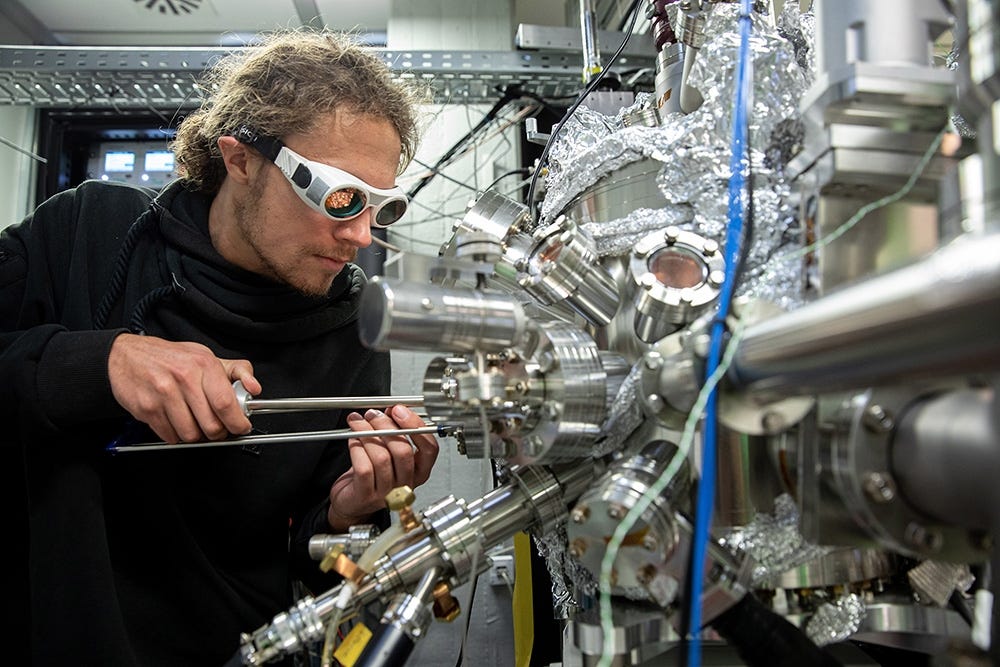
Stuttgart scientist working on a sample in the ultrafast scanning tunneling microscope. (CREDIT: University of Stuttgart)
"With the method we developed, you can make things visible that no one has seen before," says Prof. Sebastian Loth, Managing Director of the Institute for Functional Matter and Quantum Technologies at the University of Stuttgart.
"This makes it possible to settle questions about the movement of electrons in solids that have been unanswered since the 1980s." The findings of Loth's group have significant practical implications for developing new materials.
Tiny Changes with Macroscopic Consequences
In basic materials like metals, insulators, and semiconductors, altering a few atoms doesn't affect their overall properties. For instance, modified metals still conduct electricity, and insulators remain non-conductive.
However, in advanced, lab-produced materials, minimal atomic changes can result in entirely new macroscopic behaviors. Some materials can transition from insulators to superconductors, conducting electricity without heat loss. These changes occur in picoseconds—a trillionth of a second, an incredibly brief time span.
Loth's team has discovered a method to observe material behavior during these tiny atomic changes. They studied a niobium and selenium compound to observe the collective motion of electrons in a charge density wave. By applying a one-picosecond electrical pulse, the researchers could disturb this wave, creating nanometer-sized distortions in the electron collective, leading to complex electron movement for a brief period.
Preliminary work was conducted at the Max Planck Institute for Solid State Research and the Max Planck Institute for the Structure and Dynamics of Matter, where Loth previously researched.
Understanding how the electron collective movement is halted allows for the targeted development of materials with specific properties. Loth explains, "If we can understand how the movement of the electron collective is stopped, then we can also develop materials with desired properties in a more targeted manner."
The microscopy method helps to understand impurity arrangement to achieve desired technical effects. "Design at the atomic level has a direct impact on the macroscopic properties of the material," Loth notes. This understanding could lead to ultra-fast switching materials for future sensors or electronic components.
"Established methods for visualizing individual atoms or their movements either achieve high spatial resolution or high temporal resolution," explains Loth. To achieve both, his team combined a scanning tunneling microscope, which resolves materials at the atomic level, with ultrafast spectroscopy known as pump-probe spectroscopy.
The laboratory setup must be extremely well shielded to take these measurements. Vibrations, noise, air movement, and fluctuations in temperature and humidity can all interfere. "We measure extremely weak signals that are otherwise easily lost in the background noise," Loth points out. The team optimized their microscope to repeat the experiment 41 million times per second, achieving particularly high signal quality. "Only we have managed to do this so far," Loth says.
Implications for Future Research and Technology
This breakthrough allows you to observe previously invisible phenomena and helps in the development of materials with precise properties. By understanding the interaction of impurities at the atomic level, you can design materials that perform specific functions more effectively. The ability to observe electron movements in such detail opens new avenues for research in quantum technologies and material science.
You now have a powerful tool to push the boundaries of material science, potentially leading to innovations in electronics, superconductors, and other advanced technologies. This method's ability to combine high spatial and temporal resolution sets a new standard for microscopy, providing insights that were previously out of reach.
Loth's team's advancements in microscopy offer a new perspective on material behavior at the atomic level. By combining high-resolution imaging techniques, you can explore the intricate dance of electrons, paving the way for the next generation of material science discoveries.
Research findings were published in Nature Physics.
Note: Materials provided above by the The Brighter Side of News. Content may be edited for style and length.
Like these kind of feel good stories? Get the Brighter Side of News' newsletter.

Joshua Shavit
Science & Technology Writer | AI and Robotics Reporter
Joshua Shavit is a Los Angeles-based science and technology writer with a passion for exploring the breakthroughs shaping the future. As a contributor to The Brighter Side of News, he focuses on positive and transformative advancements in AI, technology, physics, engineering, robotics and space science. Joshua is currently working towards a Bachelor of Science in Business Administration at the University of California, Berkeley. He combines his academic background with a talent for storytelling, making complex scientific discoveries engaging and accessible. His work highlights the innovators behind the ideas, bringing readers closer to the people driving progress.