Schrödinger’s cat is helping quantum engineers detect errors
Scientists create robust Schrödinger cat states in antimony nuclei, advancing error-tolerant quantum computing with scalable silicon technology.
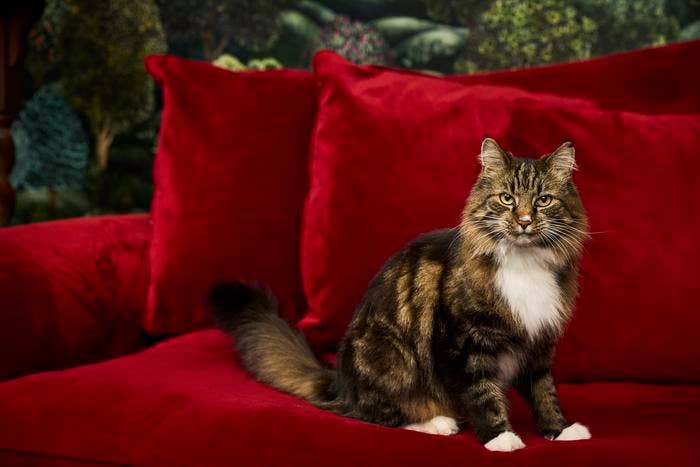
Researchers have demonstrated Schrödinger cat states in antimony nuclei, paving the way for error-resistant, scalable quantum computing in silicon chips. (CREDIT: UNSW Sydney)
Quantum computing continues to evolve, pushing the boundaries of science and technology. A groundbreaking study has now taken inspiration from the famous Schrödinger’s cat thought experiment to create a new approach to error-tolerant quantum computation.
Researchers from UNSW Sydney, the University of Melbourne, and other institutions have demonstrated the creation and manipulation of Schrödinger cat states in an antimony atom, opening new doors for scalable and robust quantum technologies.
Schrödinger’s cat, a famous metaphor in quantum mechanics, describes a scenario where a cat is simultaneously alive and dead, based on the superposition of quantum states. While no physical cat exists in this dual state, the concept highlights the peculiar nature of quantum superpositions.
UNSW’s Professor Andrea Morello explains, “People use the Schrödinger’s cat metaphor to describe a superposition of quantum states that differ significantly.”
For this study, published in the journal, Nature Physics, the team used an antimony atom—a far more complex system than typical quantum bits, or qubits. Lead researcher Xi Yu notes, “In our work, the ‘cat’ is an atom of antimony. Its large nuclear spin can take eight different directions, enabling complex superposition states.”
Unlike traditional qubits, which operate on two states (“0” and “1”), the antimony nucleus’s eight states provide greater resistance to logical errors. Co-author Benjamin Wilhelm emphasizes, “In a simple qubit system, a single error can flip a ‘0’ to a ‘1’. But in the antimony system, multiple errors are needed to cause a complete state change.”
Creating and controlling these advanced quantum states required sophisticated techniques. The researchers used SU(8) and SU(2) operations within a generalized rotating frame (GRF) to manipulate the spin states of the antimony nucleus. This approach allowed for precise control of the atom’s quantum state, minimizing errors and preserving coherence.
The process began with the preparation of the nucleus in a high-spin state. By applying finely tuned nuclear magnetic resonance (NMR) pulses, the team induced covariant rotations, ensuring the quantum states evolved predictably. These operations maintained the symmetry of the system and preserved the shape of the Wigner function, a critical factor in demonstrating quantum coherence.
Key to the success of this work was the use of a multi-frequency control scheme. This enabled the researchers to perform complex rotations and create the desired Schrödinger cat states. As the experiment progressed, the increasing number of interference fringes in the Wigner function provided visual confirmation of the superposition states.
The researchers also demonstrated the use of covariant Rabi oscillations to monitor the evolution of the spin states. These oscillations, characterized by a predictable frequency and behavior, confirmed the system’s stability and precision.
Related Stories
The Wigner function snapshots at different stages of the experiment revealed the smooth progression of the spin states. By maintaining symmetry and coherence, the team was able to produce robust quantum states capable of supporting logical qubits.
One of the most significant outcomes of this research is its potential impact on quantum error correction. Quantum systems are notoriously fragile, with even minor disturbances leading to errors. However, the unique properties of the antimony nucleus’s spin states offer a natural buffer against errors.
Prof. Morello elaborates, “If the ‘0’ state represents a ‘dead cat’ and the ‘1’ state an ‘alive cat,’ a single error isn’t enough to completely flip the state. It would take seven consecutive errors to cause a full change.” This resilience makes the antimony-based system a promising platform for developing error-correctable quantum computers.
The team’s work also demonstrated error detection capabilities. By monitoring the quantum states in real time, researchers could identify and address errors before they accumulated. Yu adds, “It’s like seeing our metaphorical cat return home with a scratch. We know it got into a fight and can intervene before further damage occurs.”
The reconstructed density matrix of the antimony nucleus provided valuable insights into the quantum states. By using maximum-likelihood estimation quantum state tomography, the team achieved high-fidelity results, confirming the accuracy of their control methods. This level of precision is critical for implementing reliable quantum error correction.
The integration of antimony atoms into silicon chips marks a significant step toward scalable quantum technology. Dr. Danielle Holmes, who fabricated the chips, highlights the benefits: “Hosting the atomic ‘Schrödinger cat’ inside a silicon chip allows for exquisite control over its quantum state. Moreover, silicon-based platforms can leverage existing semiconductor manufacturing techniques, paving the way for scalable quantum devices.”
This scalability is crucial for the future of quantum computing. While current quantum computers are limited in size and functionality, the ability to integrate high-dimensional qudits into standard silicon chips offers a path to larger, more powerful systems. These advancements could eventually lead to practical quantum computers capable of solving problems beyond the reach of classical machines.
The collaborative nature of this project underscores the global effort required to advance quantum science. Researchers from Sandia National Laboratories, NASA Ames, and the University of Calgary provided critical theoretical insights, while experimental work was carried out in Australia. Prof. Morello comments, “This work exemplifies open-borders collaboration between world-leading teams with complementary expertise.”
The researchers also demonstrated the utility of their method for other high-dimensional quantum systems. By extending their techniques to different nuclear spins and materials, they envision a broad range of applications, from quantum simulation to secure communication.
The demonstration of Schrödinger cat states in an antimony nucleus represents a major milestone in quantum computing. By combining advanced control techniques with robust error tolerance, the research addresses some of the field’s most significant challenges. The next goal for the team is to demonstrate full quantum error correction, a crucial step toward realizing practical quantum computers.
“This work opens the door to a new way of performing quantum computations,” says Prof. Morello. “The information is still encoded in binary, but there is more room for error. By detecting and correcting errors early, we can ensure the integrity of the quantum code.”
The Wigner function’s role in this research cannot be overstated. As a tool for visualizing quantum states, it provided clear evidence of the superposition and coherence of the antimony nucleus. This innovation paves the way for future studies on the behavior of high-dimensional quantum systems.
As quantum computing moves closer to practical applications, breakthroughs like this bring us closer to a future where quantum technologies transform industries ranging from cryptography to materials science. The ability to reliably create and control non-classical states within scalable platforms is a game-changer for the field.
The demonstration of Schrödinger cat states in a silicon-compatible system underscores the potential for practical, manufacturable quantum computers. With continued advancements, the dream of error-tolerant quantum computation may soon become a reality.
Note: Materials provided above by The Brighter Side of News. Content may be edited for style and length.
Like these kind of feel good stories? Get The Brighter Side of News' newsletter.
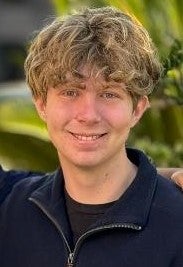