Quantum walks: What they are and how they can change the world
Quantum walk computing uses the power of quantum mechanics to solve tough problems classical computers can’t. Here’s how it works and why it matters.
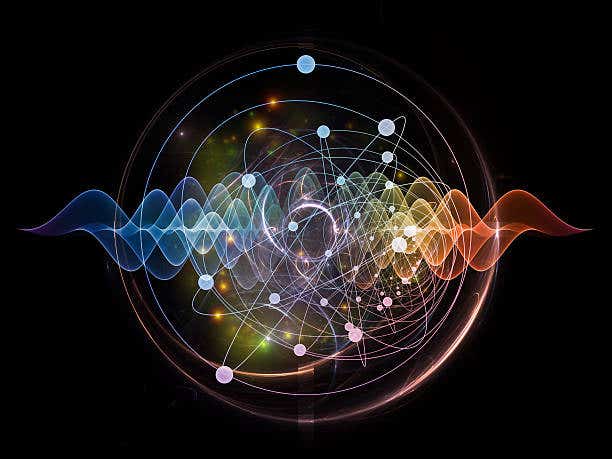
Quantum walks harness quantum physics for faster computing and problem-solving beyond classical limits. (CREDIT: iStock)
Quantum walks are changing the way scientists think about computation. They use the strange and powerful rules of quantum physics—such as superposition, interference, and entanglement—to solve problems faster and more efficiently than classical computers.
Instead of following one path at a time like classical random walks, quantum walks can explore many paths at once. This behavior opens new possibilities in designing faster algorithms, simulating complex systems, and advancing quantum computing.
A recent review by researchers from the National Innovation Institute of Defense Technology highlights the rising importance of quantum walk computing. Their paper, published in Intelligent Computing, explores how quantum walks are built, how they work, and why they matter.
How Quantum Walks Work
At their core, quantum walks are the quantum version of classical random walks. In a classical walk, a particle moves step-by-step, choosing each direction randomly. But in a quantum walk, the particle exists in many states at once. It can “walk” in several directions at the same time, thanks to superposition. When these paths interact through interference, the result is a walk that spreads out faster than any classical version.
There are several types of quantum walks. The most common are discrete-time and continuous-time models.
In discrete-time quantum walks, movement happens in steps without considering real time. These walks can use a "coin" to determine the particle’s direction—like the Hadamard or Grover models. Some don’t need a coin at all. The Szegedy and staggered models fall into this category and work well with certain graph-based structures.
Continuous-time quantum walks, on the other hand, let the particle move through a network without breaks. They use time-independent Hamiltonians to guide motion, which helps with problems involving searching and graph traversal.
There are also newer models. Discontinuous quantum walks blend features from both discrete and continuous models. They are powerful enough to support universal quantum computation by perfectly transferring quantum states across systems.
Meanwhile, nonunitary quantum walks, such as stochastic (randomly determined) and open quantum walks, act more like open quantum systems. These are useful in simulating real-world processes like photosynthesis and quantum Markov chains.
Related Stories
All types of quantum walks share one key feature—they spread out more quickly than classical walks and explore options more thoroughly. This makes them especially useful for sampling and solving problems that stump classical machines.
Building Quantum Walks in the Real World
Implementing quantum walks physically isn’t easy. Scientists use two main methods: analog and digital physical simulation.
Analog physical simulation involves creating systems—such as solid-state or optical setups—that behave like a quantum walk without translating it into a circuit. These setups can be scaled up by adding more particles or expanding the space in which they move. However, they usually lack error correction and are harder to control when simulating large or complex graphs.
Digital physical simulation, on the other hand, uses quantum circuits to model quantum walks. These circuits can include error correction and can simulate a wider range of systems. The downside is that building efficient circuits for quantum walks is still a major technical challenge. Despite that, digital approaches may offer more reliable speedups and more flexible graph simulations.
What Quantum Walks Can Do
Quantum walks are not just a scientific curiosity. They have already shown their potential in four major fields: quantum computing, quantum simulation, quantum information processing, and graph analysis.
In quantum computing, quantum walks can perform universal quantum computation. That means any calculation a quantum computer can do, a quantum walk can handle. This includes tough algebraic problems and tasks in number theory. Researchers are also exploring how quantum walks can help in machine learning and in solving optimization problems more efficiently.
Quantum simulation is another key area. Quantum walks can model quantum systems that are too complex or unstable to study in the lab. Scientists can use them to simulate multi-particle systems, predict complex physical behaviors, or understand biochemical processes at the quantum level.
In quantum information processing, quantum walks help prepare and manipulate quantum states. They’re also useful for secure communication. Their ability to move information without loss or noise makes them attractive for building safer networks and developing advanced encryption.
Graph-theoretic applications show up in network science, computer science, and data analysis. Because quantum walks naturally follow graph structures, they can uncover hidden features in complex networks. For example, they can rank nodes by importance or spot subtle differences between similar graphs. These methods are already being tested in areas like internet search, social networks, and biological systems.
Tackling Challenges Ahead
While progress is impressive, quantum walk computing still faces some major hurdles.
First, creating better algorithms remains a key challenge. Not every problem fits neatly into the quantum walk model. Designing flexible, efficient, and broadly useful algorithms is still an ongoing task.
Second, scaling up the physical systems is difficult. As the number of particles and dimensions increases, the system becomes harder to control. Researchers must find ways to build larger, more stable quantum walk setups that still perform as expected.
Finally, implementing reliable error correction and achieving fault tolerance in quantum walks is a must. Without these features, results can be unstable or inaccurate, limiting the usefulness of the technology in practical applications.
Still, each of these challenges offers direction for the future. The more we learn about quantum walks, the closer we get to building practical quantum computers. As one researcher noted, “Quantum walks not only have evolutionary merits, but also improve sampling efficiency, solving problems previously considered computationally difficult for classical systems.”
Quantum Walks Point Toward the Future
Quantum walk computing is more than just a theoretical concept. With roots in physics and branches reaching into computing, biology, and mathematics, it represents a powerful tool for solving real-world problems. As research continues and technology matures, quantum walks could become a central feature in tomorrow’s computing landscape.
They offer a unique path forward, especially in the noisy intermediate-scale quantum era, where classical models begin to fail but full quantum machines are not yet ready. By designing systems and algorithms around quantum walk principles, scientists can explore a new world of computation, one step—or quantum leap—at a time.
Note: The article above provided above by The Brighter Side of News.
Like these kind of feel good stories? Get The Brighter Side of News' newsletter.
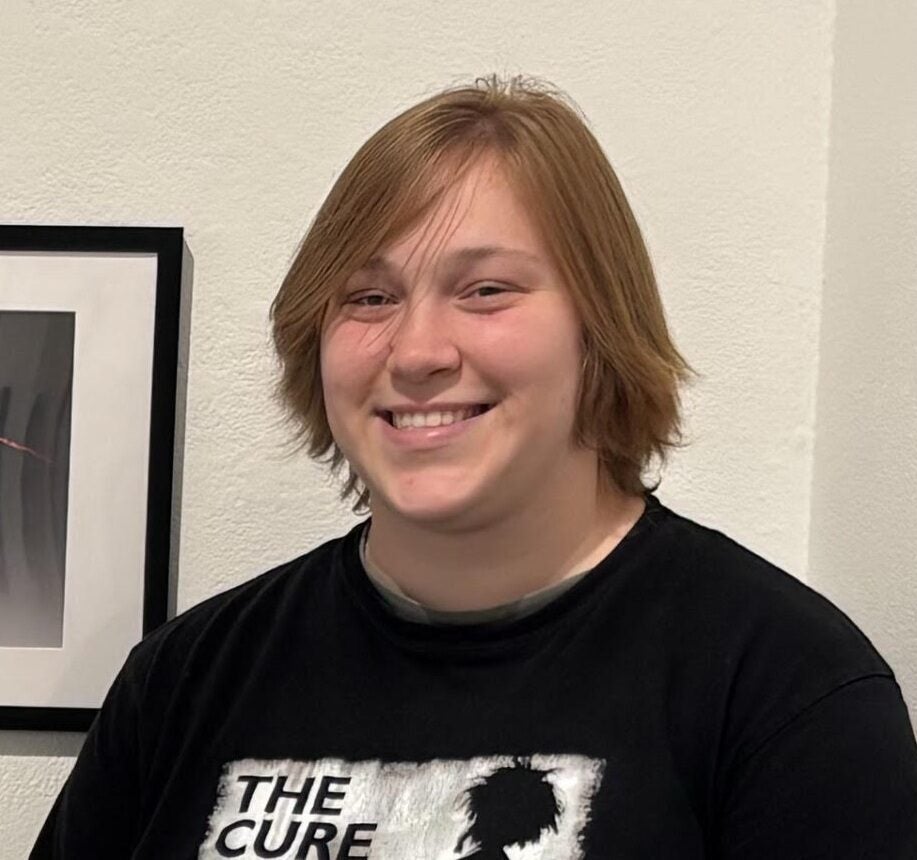
Mac Oliveau
Science & Technology Writer | AI and Robotics Reporter
Mac Oliveau is a Los Angeles–based science and technology journalist for The Brighter Side of News, an online publication focused on uplifting, transformative stories from around the globe. Passionate about spotlighting groundbreaking discoveries and innovations, Mac covers a broad spectrum of topics—from medical breakthroughs and artificial intelligence to green tech and archeology. With a talent for making complex science clear and compelling, they connect readers to the advancements shaping a brighter, more hopeful future.