Quantum sensing technology opens new doors in drug development
Quantum sensors revolutionize molecular analysis, enabling single-nucleus detection with unprecedented precision.
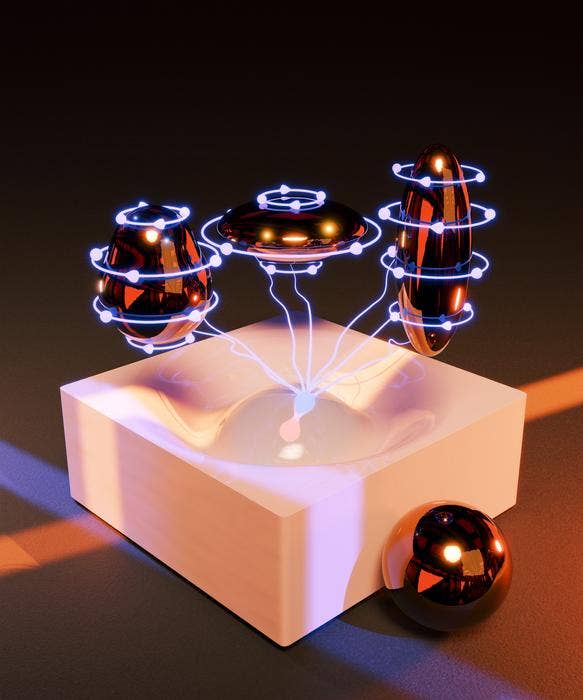
Quantum sensors embedded in diamonds are transforming molecular analysis, achieving single-nucleus detection and uncovering hidden molecular variations.
The ability to probe the smallest building blocks of matter has long fascinated scientists, fueling advancements in medicine, security, and materials science. Traditional methods like nuclear quadrupolar resonance (NQR) spectroscopy have revealed molecular structures by detecting interactions between nuclear quadrupole moments and local electric field gradients.
Despite their utility, these techniques are fundamentally limited by their reliance on macroscopic ensembles of nuclei. This limitation masks the molecule-to-molecule variations critical in fields such as protein research or drug development.
Recent breakthroughs at the University of Pennsylvania’s School of Engineering and Applied Science (Penn Engineering) have overcome these limitations. Using quantum sensors embedded in diamonds, researchers have refined NQR spectroscopy to detect signals from individual nuclei—a feat previously deemed impossible. This advancement opens the door to transformative discoveries in molecular science.
Traditional NQR spectroscopy employs radio waves to identify molecular "fingerprints," making it a staple in detecting explosives, analyzing pharmaceuticals, and conducting thermometric studies. These methods, however, average signals across trillions of atoms, overlooking subtle but significant molecular differences. In protein research, for example, small structural variations dictate functionality and can differentiate health from disease.
Quantum sensors based on defects in diamond—specifically nitrogen-vacancy (NV) centers—now offer a solution. NV centers, atomic-scale imperfections in the diamond lattice, can detect individual nuclear spins. These centers host electronic spin states that are manipulated with laser light and microwave signals at room temperature. B
y using advanced control sequences like Dynamical Decoupling (DD), researchers amplify hyperfine couplings, enabling unprecedented sensitivity and precision.
“This technique allows us to isolate individual nuclei and reveal tiny differences in what were thought to be identical molecules,” explains Lee Bassett, director of Penn’s Quantum Engineering Laboratory and senior author of the study published in Nano Letters. “By focusing on a single nucleus, we can uncover details about molecular structure and dynamics that were previously hidden.”
The breakthrough emerged from experiments involving NV centers in diamonds. Alex Breitweiser, then a doctoral student at Penn and co-first author of the study, observed unexpected periodic signals during routine experiments. Initially dismissed as artifacts, the patterns persisted despite rigorous troubleshooting. Returning to decades-old nuclear magnetic resonance textbooks, Breitweiser identified a previously overlooked physical mechanism.
Related Stories
Advances in quantum sensing technologies enabled the detection of effects once considered insignificant. “We realized we weren’t just seeing an anomaly,” Breitweiser recalls. “We were breaking into a new regime of physics that we can access with this technology.”
Further collaboration with Delft University of Technology refined the method. By integrating experimental physics, quantum sensing, and theoretical modeling, the team developed a tool capable of capturing single-atomic signals with extraordinary precision. “This is a bit like isolating a single row in a huge spreadsheet,” explains Mathieu Ouellet, another co-first author. “Traditional NQR provides an average, but we’ve isolated unique data points from individual nuclei.”
At the heart of this discovery lies the NV center’s interaction with its intrinsic nitrogen-14 nuclei. The electronic spin of the NV center interacts with nearby nuclear spins, including nitrogen-14 and other isotopes like carbon-13.
The researchers leveraged these interactions to map the nuclear quadrupolar Hamiltonian—a mathematical representation of the system’s quantum behavior. They observed significant variations in quadrupolar and hyperfine parameters between NV centers, as well as a previously unreported symmetry-breaking quadrupolar term.
The experiments also revealed correlations between nuclear Hamiltonian parameters and electronic zero-field splitting (ZFS) parameters, shedding light on local chemical structures and deformations caused by electric or strain fields. These findings underscore the potential of quantum-enhanced NQR spectroscopy to explore previously hidden aspects of molecular behavior.
Ouellet’s efforts to decode these findings involved extensive simulations and hypothesis testing. “The data pointed to something unusual, but there were multiple potential explanations,” he notes. “It took significant effort to arrive at the correct diagnosis.”
The ability to measure these parameters with such precision also opens new avenues for understanding the effects of strain and electric fields on molecular structures.
Researchers envision a future where these quantum techniques can be applied to study the mechanics of protein folding or the interactions between drugs and their molecular targets. Such studies are essential for developing next-generation pharmaceuticals and understanding diseases at the molecular level.
The implications of this research extend far beyond molecular characterization. By enabling precise control and measurement of individual nuclei, quantum-enhanced NQR spectroscopy could revolutionize drug development, protein analysis, and materials science.
For example, studying molecule-to-molecule variations could provide insights into dynamic processes like protein folding or drug-target interactions, paving the way for breakthroughs in personalized medicine.
The team’s ability to isolate and manipulate nuclear states at room temperature also hints at broader applications in quantum computing and sensing technologies. As Bassett puts it, “We are studying the building blocks of the natural world at an entirely new scale.”
The method’s sensitivity to local chemical environments could also advance the study of materials under extreme conditions, such as high pressure or temperature. These insights would be invaluable for designing materials with specific properties, such as superconductors or advanced catalysts for renewable energy applications.
This research not only demonstrates the power of quantum sensors but also highlights the collaborative nature of modern science. Partnerships between institutions, including those at Delft University of Technology, were instrumental in pushing the boundaries of what is possible. By combining theoretical expertise with experimental innovation, the team has set the stage for a new era in molecular analysis.
By characterizing phenomena previously hidden from view, this innovation offers a powerful new lens for understanding the molecular mechanisms that govern life and matter.
It exemplifies how quantum technologies can push the boundaries of scientific inquiry, promising a future where precision and depth of understanding are no longer limited by the scale of observation.
Note: Materials provided above by The Brighter Side of News. Content may be edited for style and length.
Like these kind of feel good stories? Get The Brighter Side of News' newsletter.