Quantum breakthrough: Scientists created antimatter from light
Scientists have simulated gamma-ray collisions, producing antimatter jets and unlocking new insights into extreme astrophysical phenomena.
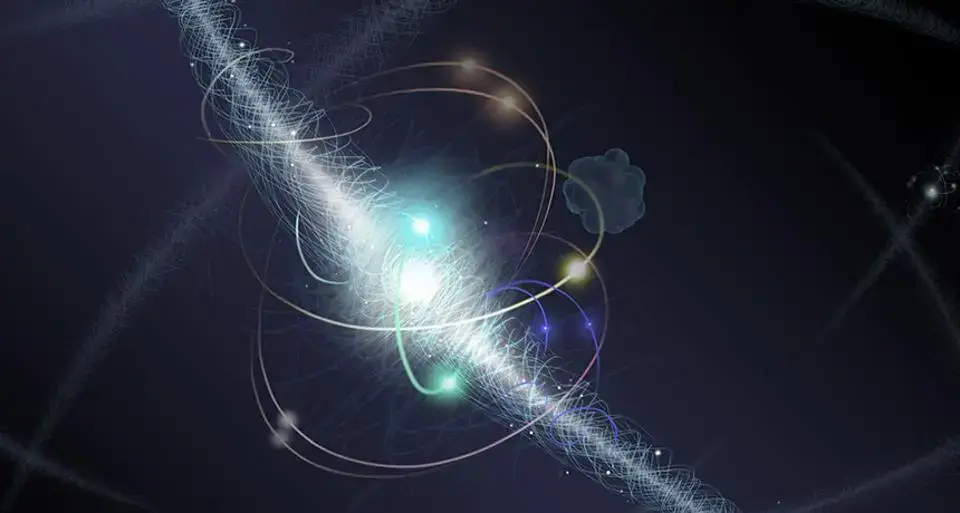
High-intensity lasers now enable scientists to create antimatter jets in the lab. (CREDIT: Nicole Rager Fuller/NSF)
High-powered lasers have transformed the way researchers explore extreme physical phenomena, pushing the boundaries of quantum physics and astrophysics. These lasers, when focused to nearly their diffraction limit, generate electromagnetic fields of immense strength.
Such fields not only allow the acceleration of energetic particles but also enable the study of processes that typically occur only in the most extreme environments, such as the creation of electron-positron pairs—a phenomenon previously confined to astrophysical settings like pulsar magnetospheres.
At laser intensities exceeding 10²³ W/cm², quantum-electrodynamical (QED) effects become non-linear. One of the most fascinating processes is the Breit-Wheeler process, in which gamma photons collide to produce matter-antimatter pairs, specifically electrons and positrons.
While non-linear pair creation using intense lasers has been observed, the linear Breit-Wheeler process, which requires two real photons to interact, remains elusive in laboratory settings. This process is rare due to its small cross-section, demanding high photon fluxes for successful observation.
Creating these conditions involves innovative experimental setups. High-intensity laser facilities such as the Extreme Light Infrastructure Beamlines and Apollon offer unprecedented opportunities to explore these regimes. These facilities enable dense flashes of gamma rays through colliding laser pulses, simulating astrophysical conditions.
Recent advances in micrometer-scale structured plasma targets have further opened doors to achieving photon collisions that produce electron-positron pairs.
Innovative Experimental Configurations
A breakthrough concept, proposed by a team led by Yutong He of the University of California, San Diego, demonstrates how structured plasma targets irradiated by lasers can create conditions where the linear Breit-Wheeler process dominates.
Related Stories
The experimental setup involves a plastic block etched with micrometer-scale crisscrossing channels. High-powered lasers strike this block from opposite sides, generating high-energy electron clouds that accelerate toward each other. These accelerated electrons emit gamma photons upon collision, which then collide with each other to produce electron-positron pairs.
“When the laser pulses penetrate the sample, each of them accelerates a cloud of extremely fast electrons,” explained Toma Toncian of Helmholtz-Zentrum Dresden-Rossendorf in Germany. “These two electron clouds then race toward each other with full force, interacting with the laser propagating in the opposite direction.”
The generated gamma photons collide, initiating the Breit-Wheeler process. Even more remarkably, the collision creates magnetic fields that confine and accelerate the positrons into narrow, jet-shaped beams. Within a distance of just 50 micrometers, the positrons can achieve energy levels of up to one gigaelectronvolt.
Astrophysical Implications in the Lab
This experimental setup mimics processes thought to occur near neutron stars, specifically pulsars. These rapidly spinning, magnetized stars emit collimated jets of particles, believed to result from gamma-ray collisions in their magnetospheres.
According to Alexey Arefiev of UC San Diego, “With our new concept, such phenomena could be simulated in the laboratory, at least to some extent, which would then allow us to understand them better.”
These findings bridge the gap between laboratory physics and astrophysical phenomena. By studying these processes in controlled settings, scientists can gain insights into the extreme environments surrounding neutron stars and the mechanisms that populate their magnetospheres with plasma.
The balance between linear and non-linear pair creation in these environments is crucial, as it dictates the density and dynamics of plasma near pulsars.
Future Prospects
The promise of this experimental design extends beyond mere observation of the Breit-Wheeler process. The ability to confine and accelerate positrons has implications for generating antimatter jets, which could serve as a model for understanding astrophysical jet dynamics.
Preliminary tests at the European XFEL X-ray laser facility aim to validate predictions about the magnetic fields generated during these experiments.
Furthermore, these experiments align well with the capabilities of the Extreme Light Infrastructure Nuclear Physics facility in Romania, which features advanced short-pulse lasers and gamma-ray beams. This facility’s unique infrastructure is perfectly suited for exploring high-intensity laser-matter interactions and validating theoretical predictions.
The significance of this work is underscored by the sheer scale of the positron yield. Simulations suggest that at laser intensities below 5 × 10²² W/cm², the positron production rate could reach ~10⁹, a staggering four orders of magnitude greater than previous proposals.
Such advancements offer a unique opportunity to study not just the Breit-Wheeler process but also the transition between linear and non-linear pair cascades.
The researchers’ findings, published in Communications Physics, demonstrate that current laser technology is sufficient to achieve these groundbreaking results. This realization propels high-intensity laser experiments into a new era, where laboratory-based studies of astrophysical phenomena become increasingly feasible.
As these methods evolve, they hold the potential to unlock deeper understanding of the universe's most extreme environments and the fundamental laws that govern them.
Note: Materials provided above by The Brighter Side of News. Content may be edited for style and length.
Like these kind of feel good stories? Get The Brighter Side of News' newsletter.
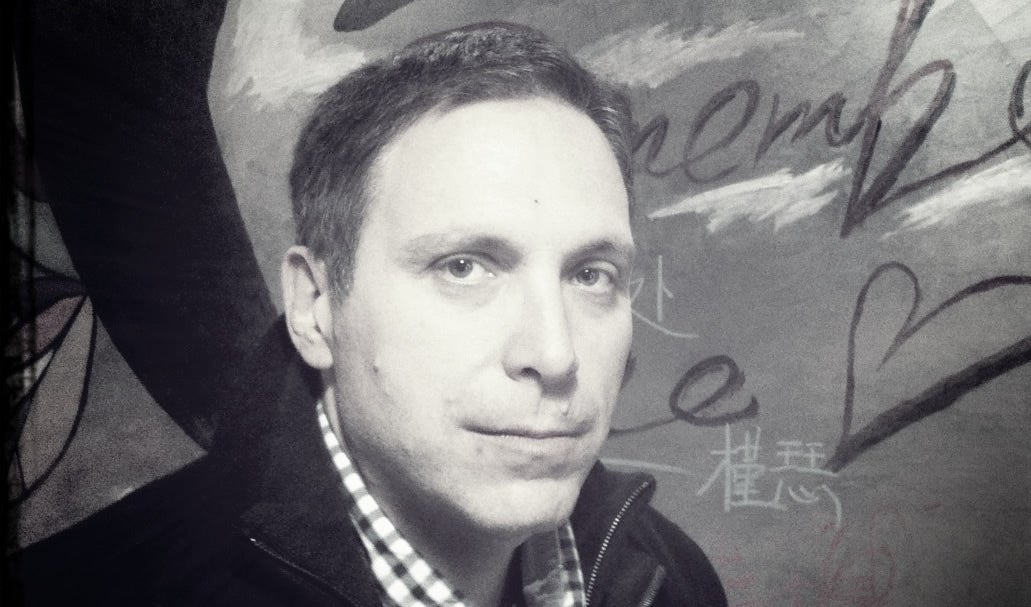