Physicists develop tiny atomic compasses to improve navigation and brain imaging
Scientists developed atomic sensors that precisely measure magnetic field direction, paving the way for advancements in navigation and neuroscience.
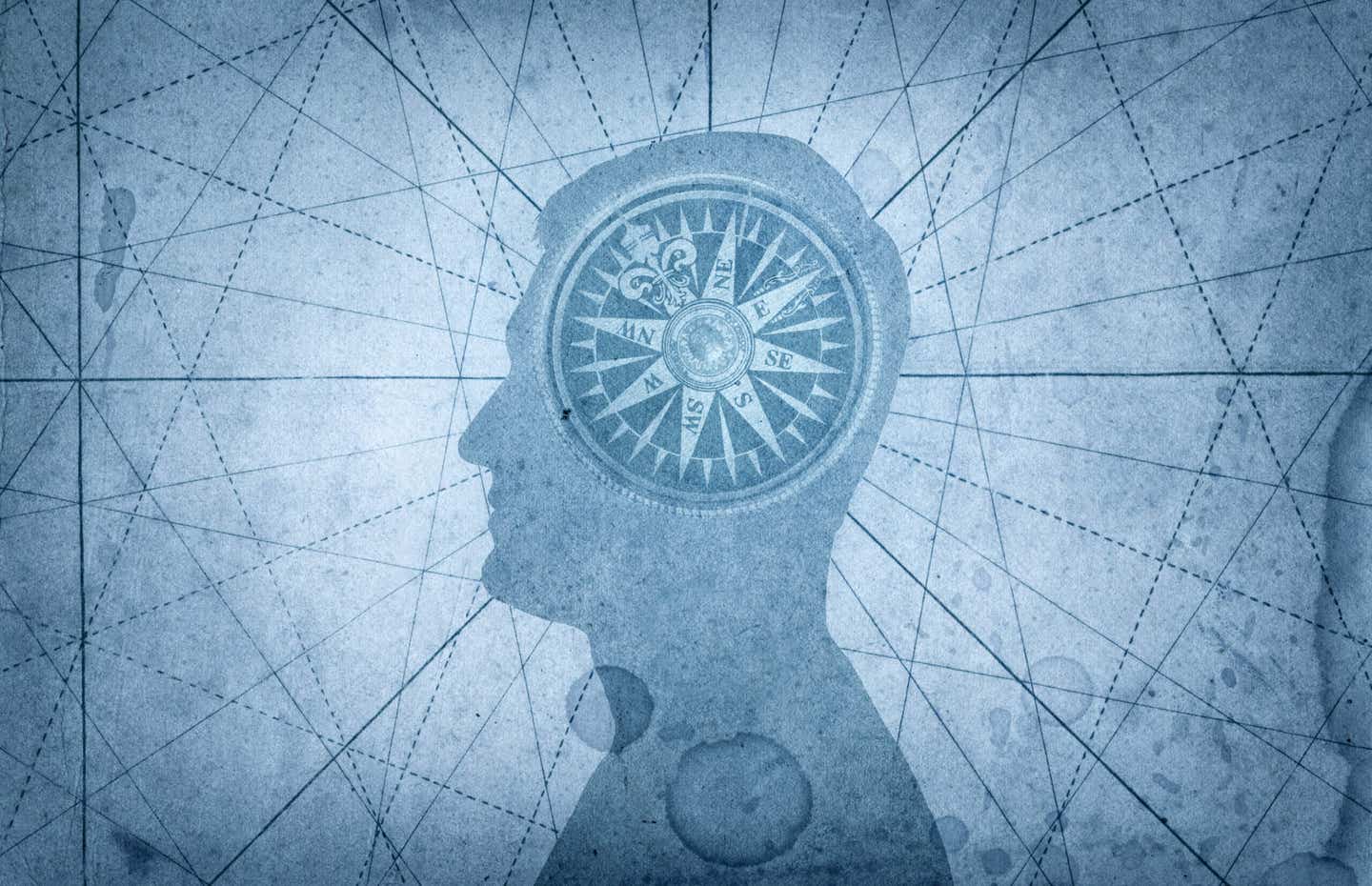
Researchers at CU Boulder have developed atomic sensors that measure magnetic field direction with unprecedented accuracy. (CREDIT: CC BY-SA 4.0)
Physicists and engineers have developed a groundbreaking method to measure magnetic field orientation using atoms as tiny compasses. This advancement could enhance applications in quantum sensing, brain imaging, and navigation.
Researchers at CU Boulder have demonstrated a new way to use optically pumped magnetometers (OPMs) to improve directional accuracy in magnetic field measurements.
Magnetic fields surround us. Earth’s iron-rich core generates a massive magnetic field that shields the planet. The human brain produces small magnetic pulses when neurons fire. Detecting these fields has long been possible, but precisely measuring their direction has remained a challenge.
Traditional magnetometers like superconducting quantum interference devices (SQUIDs) and fluxgates measure specific magnetic field components but require careful calibration and shielding from external interference.
OPMs, based on atomic spin precession, offer a more precise alternative by responding only to the total magnetic field strength, independent of sensor orientation. This has made them useful for applications such as biosensing, contraband detection, and dark matter searches. However, they struggle with vector accuracy, which is essential for navigation, space exploration, and geophysical studies.
The Challenge of Vector Magnetometry
Vector magnetometry requires knowing not only the strength of a magnetic field but also its exact direction. This information is crucial for applications like planetary exploration, where accurately mapping a planet’s internal magnetic field can reveal insights into its composition and history.
Current vector OPMs rely on external reference fields generated by coil systems. These coils create controlled magnetic fields that help determine the sensor’s orientation. However, this approach has limitations.
Coil systems introduce physical complexity, require frequent recalibrations, and can drift over time. They also struggle with environmental influences such as temperature fluctuations and mechanical wear, which degrade accuracy.
One alternative involves using electromagnetic waves as a reference. By coupling atomic ensembles to the polarization of these waves, scientists can extract vector information without relying on external magnetic fields.
Related Stories
Techniques such as electromagnetically induced transparency (EIT) and nonlinear magneto-optical rotation (NMOR) allow for precise, remote detection of magnetic field directions. Unlike coil-based methods, these approaches do not degrade in high magnetic fields and can be miniaturized more easily.
Atoms as Tiny Compasses
A research team at CU Boulder, led by physicists Cindy Regal and Svenja Knappe, has taken a major step forward in solving these challenges. Their work, published in Optica, demonstrates how atoms in a vapor can function as highly sensitive directional magnetometers.
“Atoms can tell you a lot,” Regal explained. “We’re data mining them to glean simultaneously whether magnetic fields are changing by extremely small amounts and what direction those fields point.”
The team used a chamber containing about a hundred billion rubidium atoms in vapor form. When exposed to a magnetic field, these atoms experienced energy shifts. By shining a laser through the chamber, the researchers measured how the atoms responded, revealing the field’s precise orientation.
“You can think of each atom as a compass needle,” said Dawson Hewatt, a graduate student at JILA. “And we have a billion compass needles, which could make for really precise measurement devices.”
Unlike mechanical sensors, which can wear down over time, atomic sensors remain stable. Their behavior is governed by fundamental quantum mechanics, making them highly reliable.
Real-World Applications
Knappe, a quantum engineer specializing in magnetic imaging, sees vast potential for this technology. “What magnetic imaging allows us to do is measure sources that are buried in dense and optically opaque structures,” she said. “They’re underwater. They’re buried under concrete. They’re inside your head, behind your skull.”
Knappe co-founded FieldLine Inc., a company that develops atomic vapor magnetic sensors. These sensors are small enough to fit into a helmet and can map brain activity with high precision. Current OPMs require shielded rooms to function properly, but the CU Boulder team’s research could enable sensors to work in normal environments while maintaining directional accuracy.
Medical applications are particularly promising. Understanding how magnetic fields shift inside the brain could provide insights into neurological disorders like Alzheimer’s and Parkinson’s disease. OPMs could help detect these changes earlier than traditional imaging methods.
The technology could also revolutionize navigation. Airplanes rely on GPS for positioning, but atomic sensors could allow them to navigate using Earth’s magnetic field, much like migratory birds. This approach would be particularly useful in areas where GPS signals are weak or unreliable, such as underwater or in deep space.
Instead of relying on external calibration using metal coils, the CU Boulder team used microwave antennas as a stable reference. “Ultimately, we can read out those wiggles, which tell us about the strength of the energy transitions the atoms are undergoing, which then tells us about the direction of the magnetic field,” Regal explained.
With further refinements, these atomic sensors could surpass existing vector magnetometers in both precision and stability. Some current sensors can achieve similar directional accuracy, but they require extensive recalibration. Atomic systems, on the other hand, remain fundamentally unchanged over time, making them more reliable in the long run.
The team’s next goal is to improve the precision of these atomic compasses and test them in real-world conditions. If successful, they could provide a new way for pilots, submarines, and even astronauts to navigate without relying on traditional instruments.
“It’s now a question of: ‘How far can we push these atomic systems?’” Knappe said.
Note: Materials provided above by The Brighter Side of News. Content may be edited for style and length.
Like these kind of feel good stories? Get The Brighter Side of News' newsletter.
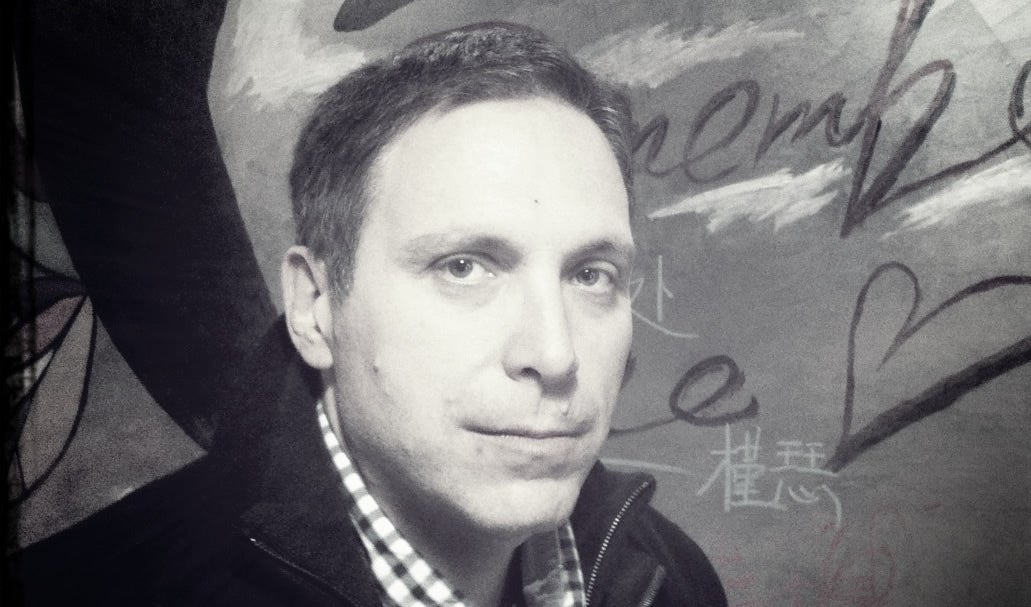
Joseph Shavit
Head Science News Writer | Communicating Innovation & Discovery
Based in Los Angeles, Joseph Shavit is an accomplished science journalist, head science news writer and co-founder at The Brighter Side of News, where he translates cutting-edge discoveries into compelling stories for a broad audience. With a strong background spanning science, business, product management, media leadership, and entrepreneurship, Joseph brings a unique perspective to science communication. His expertise allows him to uncover the intersection of technological advancements and market potential, shedding light on how groundbreaking research evolves into transformative products and industries.