New research explains how air pollution forms in the atmosphere
Discover how sulfur chemistry at the molecular level influences urban air pollution and climate, unveiling critical insights into aerosol behavior.
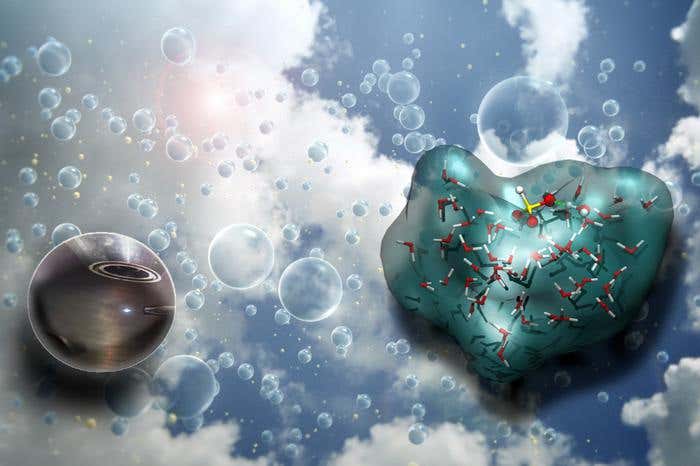
Explore groundbreaking research on sulfur chemistry, revealing its impact on air pollution and climate through detailed molecular-level studies. (CREDIT: FHI)
Understanding the intricate chemical processes of the atmosphere is key to predicting and improving air quality. Among these processes, the sulfur cycle is crucial. Sulfur enters the atmosphere from both natural and human activities, with sulfur dioxide (SO2) being the primary contributor. The concentration of SO2 varies significantly by region, influencing local air quality and environmental health.
Sulfuric acid (H2SO4), derived from SO2, plays a major role in acidifying the atmosphere. This acid contributes to aerosol formation and increases the acidity of rainwater and cloud droplets.
The transformation of SO2 into sulfuric acid involves reactions with hydroxyl (OH) radicals in the gas phase or other oxidants like ozone (O3), nitrogen dioxide (NO2), and hydrogen peroxide (H2O2) in aqueous environments such as aerosols and cloud droplets. These reactions are integral to the cycling of halogens and nitrogen oxides, which are vital atmospheric components and contribute to broader climatic effects.
The reactivity of these processes is influenced by the high ionic strength of aerosol particles and the pH of their surroundings. Enhanced reaction rates under these conditions often result in a rapid increase in aerosol mass, particularly during haze events in polluted urban areas. These events, characterized by reduced visibility and severe health impacts, highlight the importance of understanding sulfur chemistry at the molecular level.
The process begins with SO2 dissolving in water to form sulfurous acid (H2SO3), which then dissociates into various species like bisulfite and sulfonate, depending on conditions such as pH and temperature. These transformations are influenced by the unique properties of the liquid-vapor interface, where surface chemistry plays a dominant role.
The surface of aerosols significantly affects these reactions. Experimental evidence shows that SO2 forms surface complexes upon adsorption at the gas-aqueous interface. These complexes influence the reaction kinetics, particularly for S(IV) oxidation, which is critical for understanding haze formation.
Published in the journal, Nature Communications, recent studies also highlight that the reaction pathways at this interface differ from those in the bulk solution. These differences underscore the complexity of atmospheric chemistry and the necessity for accurate modeling to predict pollutant behavior effectively.
Researchers have focused on understanding the acid-base equilibria of these species. Investigations using spectroscopic techniques have revealed differences in the behavior of these compounds between the bulk solution and the surface. Molecular dynamics simulations support these findings, showing that the bisulfite tautomer is depleted at low pH at the liquid-vapor interface, favoring sulfonate formation instead.
Related Stories
This shift results from ion pairing between sulfonate and hydronium ions and the higher energy barrier for sulfonic acid dehydration at the interface. These findings suggest that the molecular environment at the interface significantly alters chemical behaviors, influencing the uptake and transformation of pollutants.
These molecular-level insights are essential for improving atmospheric models. The findings demonstrate that the interface—a layer narrower than a human hair—plays a significant role in determining how SO2 reacts and transforms in the atmosphere. For example, the enhanced stabilization of sulfonate at the interface affects how SO2 interacts with other atmospheric pollutants like nitrogen oxides and hydrogen peroxide.
Such interactions are crucial in urban haze formation and broader climatic impacts. This understanding helps explain the unexpected rapid growth in aerosol mass during high-pollution episodes, providing a clearer picture of the mechanisms driving such events.
The study underscores the challenges of measuring chemical equilibria at the liquid-vapor boundary compared to the bulk of a solution. However, understanding these processes at the molecular level allows for better predictions of aerosol behavior and their effects on air pollution and climate change.
This research provides valuable data to refine climate and air quality models, ultimately contributing to strategies aimed at mitigating air pollution. Improved models not only help predict the behavior of aerosols but also their interactions with sunlight and their role in cloud formation, both of which have profound implications for global climate.
Key findings from the research emphasize the distinct chemical behaviors at the interface. Under acidic conditions, sulfonate species are more stable, driven by unique stabilization mechanisms. This behavior contrasts with bulk solutions, where bisulfite predominates under similar conditions.
The enhanced stabilization at the interface also explains the faster reaction rates observed during haze events, as these conditions promote aerosol mass growth. Such insights underline the critical role of surface chemistry in driving atmospheric transformations, particularly in regions experiencing heavy pollution.
The study highlights the importance of accurate knowledge of surface equilibria and their impact on sulfur dioxide uptake and reaction kinetics. This understanding is crucial for addressing severe pollution episodes linked to haze in urban environments.
By examining these processes, scientists can develop more effective strategies to reduce the harmful impacts of air pollution. The broader implications of this research also extend to health, as exposure to pollutants like SO2 is linked to respiratory and cardiovascular issues, emphasizing the urgent need for actionable solutions.
The implications of these findings extend beyond air quality. Improved models of atmospheric chemistry help predict how aerosols influence climate patterns. For instance, aerosols affect cloud formation, reflect solar radiation, and alter precipitation patterns.
These changes have cascading effects on ecosystems, agriculture, and water resources, making the study of sulfur chemistry a cornerstone of environmental science. Understanding these processes also supports international efforts to address climate change by reducing emissions of key pollutants and improving regulations targeting industrial and vehicular sources.
Research like this sheds light on the complexities of atmospheric processes, bridging gaps in our understanding of air pollution and its broader impacts. These findings emphasize the need for continued investigation into surface chemistry, as it holds the key to addressing some of the most pressing environmental issues of our time.
The integration of experimental and computational approaches, as demonstrated in this study, provides a robust framework for future research, ensuring that scientific advancements continue to inform public policy and environmental management strategies.
Note: Materials provided above by The Brighter Side of News. Content may be edited for style and length.
Like these kind of feel good stories? Get The Brighter Side of News' newsletter.
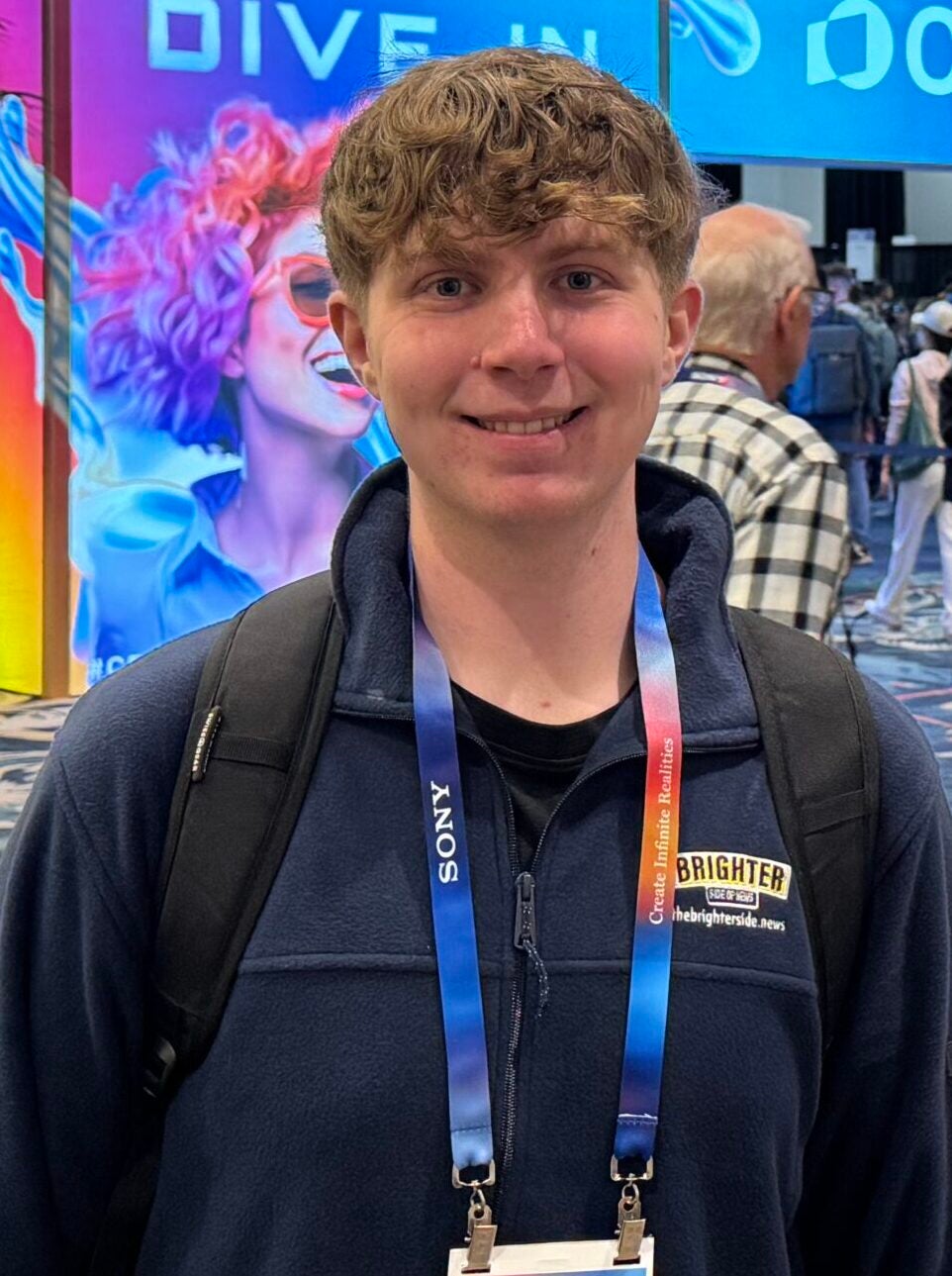
Joshua Shavit
Science & Technology Writer | AI and Robotics Reporter
Joshua Shavit is a Los Angeles-based science and technology writer with a passion for exploring the breakthroughs shaping the future. As a contributor to The Brighter Side of News, he focuses on positive and transformative advancements in AI, technology, physics, engineering, robotics and space science. Joshua is currently working towards a Bachelor of Science in Business Administration at the University of California, Berkeley. He combines his academic background with a talent for storytelling, making complex scientific discoveries engaging and accessible. His work highlights the innovators behind the ideas, bringing readers closer to the people driving progress.