New quantum clock uses entanglement to push science beyond existing limits
Quantum clocks harness entanglement to surpass precision limits, revolutionizing timekeeping and opening new frontiers in technology and science.
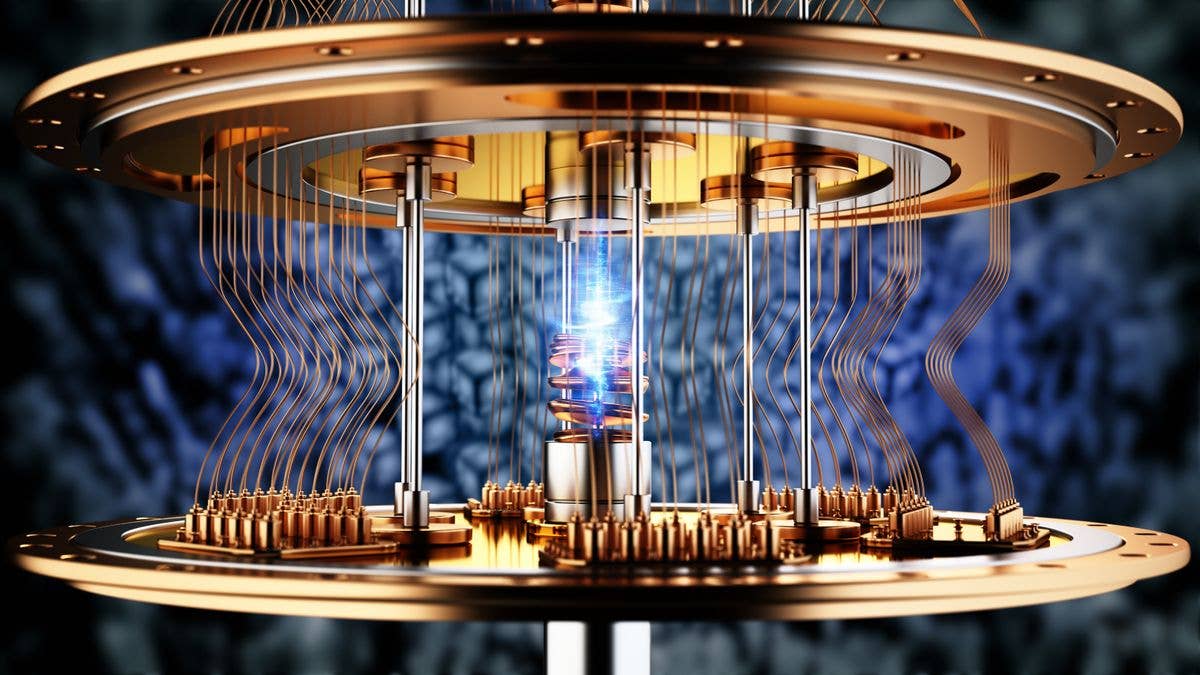
Advancements in quantum clocks leverage entanglement to surpass the standard quantum limit, paving the way for groundbreaking precision and new technologies. (CREDIT: CC BY-SA 4.0)
Quantum technologies are reshaping the future of measurement and sensing, and advancements in quantum clocks are at the forefront. These devices, which measure time based on the natural oscillations of atoms, have reached levels of precision unimaginable just a decade ago.
Recent breakthroughs highlight how quantum entanglement can push timekeeping beyond the standard quantum limit (SQL), opening doors to novel applications in science and technology.
At the heart of quantum measurements lies a fundamental challenge: quantum projection noise (QPN). This noise creates inherent uncertainty when measuring multiple identical quantum sensors, limiting precision to the SQL. The SQL dictates that the uncertainty decreases proportionally to the square root of the number of sensors.
However, quantum theory suggests a more precise boundary known as the Heisenberg limit (HL), where uncertainty scales inversely with the number of sensors.
Achieving the HL requires using entangled or non-classical quantum states. Such entanglement has demonstrated benefits in areas ranging from fundamental physics to biology. By integrating entanglement into optical atomic clocks, researchers are moving closer to these precision limits.
Quantum projection noise has been a significant barrier in various measurement applications. From nanoscale imaging to gravimetry and optical atomic clocks, achieving the HL can revolutionize how these systems operate.
In biological contexts, enhanced precision could improve imaging techniques, leading to breakthroughs in medical diagnostics. Similarly, in fundamental physics, these advancements could refine measurements of universal constants and gravitational waves.
Recent innovations, published in the journal, Nature, merge programmable atom arrays and optical atomic clocks. Programmable atom arrays, a leading architecture for quantum information processing, now boast gate fidelities as high as 99.5%.
Optical atomic clocks, capable of measuring time with fractional frequency uncertainties below 10⁻¹⁸, achieve remarkable stability when measurements are averaged over time. Combining these capabilities creates a pathway for entanglement-enhanced measurements.
One promising avenue involves tweezer-controlled optical atomic clocks. These systems can generate many-body entangled states, such as Schrödinger cat states, which are coherent superpositions of macroscopically distinct quantum states.
Related Stories
These states, exemplified by Greenberger–Horne–Zeilinger (GHZ) configurations, amplify phase sensitivity and allow measurements at the HL. However, their fragility to noise and decay presents significant challenges.
Tweezer-based systems also allow for microscopic control over individual atoms, offering a unique platform for manipulating quantum states. By leveraging these capabilities, researchers can design tailored experiments to test the limits of quantum mechanics.
For example, studies using these systems can explore the interplay between entanglement and decoherence, deepening our understanding of quantum phenomena.
A team of researchers has pioneered GHZ state generation in neutral-atom optical clocks, a first in the field. Using strontium atoms arranged in a lattice, they created entangled states of up to nine atoms. The team demonstrated fractional frequency instability below the SQL during an atom-laser comparison at a restricted dark time of three milliseconds.
To achieve this, they extended the Rydberg-gate toolkit to produce fully connected graph states. This innovation enabled them to attain raw Bell-state fidelities of 98.3% and apply GHZ states in metrology. While their setup currently limits effective operation to milliseconds, these advancements lay the groundwork for overcoming such constraints through multi-ensemble metrology.
These experiments mark a critical milestone in quantum metrology. By demonstrating below-SQL performance in a real-world scenario, the researchers have opened new possibilities for applying GHZ states in practical devices. Future experiments could involve larger atom arrays, enabling even greater entanglement and pushing the boundaries of quantum precision.
Entanglement’s unique properties make it a powerful tool for quantum systems. When particles become entangled, information about one reveals information about the other, no matter the distance between them. In clocks, entangled atoms act collectively, mimicking a single entity. This collective behavior reduces measurement uncertainty and increases precision.
For example, researchers nudged strontium atoms into “fluffy” orbits, allowing their electrons to interact. These interactions created strongly entangled pairs, which “ticked” faster than individual atoms. By incorporating combinations of individual and entangled atoms, the team effectively created a clock with multiple ticking rates.
“What we’re able to do is divide the same length of time into smaller and smaller units,” said Adam Kaufman, a senior author of the study and fellow at JILA, a joint institute of the University of Colorado Boulder and the National Institute of Standards and Technology (NIST). “That acceleration could allow us to track time more precisely.”
This concept of entanglement extends beyond clocks. In quantum communication, entangled particles could enable secure data transmission. In quantum computing, entangled qubits are essential for performing complex calculations. The implications of this technology are vast, influencing fields as diverse as cryptography and artificial intelligence.
Quantum clocks have applications far beyond telling time. Their precision can measure subtle changes in environmental conditions, such as variations in Earth’s gravitational field. By lifting an optical clock by a fraction of a millimeter, one can detect changes in gravity, making these devices invaluable in fields like geophysics and climate science.
Kaufman’s team’s methods also offer potential for advancing quantum computing. Entangling atoms in clocks mirrors operations used in quantum computers. These operations, known as multi-qubit gates, could eventually enable quantum systems to perform calculations far beyond the capabilities of classical computers.
“The question is: Can we create new kinds of clocks with tailored properties, enabled by the exquisite control that we have in these systems?” Kaufman said.
Such control could lead to breakthroughs in navigation and positioning systems. For instance, quantum clocks could enhance GPS accuracy, enabling centimeter-level precision. This advancement would benefit industries ranging from autonomous vehicles to resource exploration.
While these innovations represent significant progress, challenges remain. GHZ states, though powerful, are fragile. Prolonged operation causes entanglement to degrade, limiting their practical use. However, multi-ensemble metrology offers a potential solution by enabling phase estimation over extended intervals.
Kaufman and his colleagues envision creating clocks that leverage tailored quantum properties for unprecedented precision. These systems could achieve Heisenberg-limited performance, redefining the boundaries of timekeeping and measurement.
In addition to their scientific applications, these advancements have societal implications. Improved timekeeping could revolutionize telecommunications, synchronizing networks with unparalleled accuracy. Similarly, enhanced sensing capabilities could aid disaster prediction and resource management, addressing global challenges.
As quantum technologies evolve, the potential for transformative applications grows. From enhancing fundamental physics to enabling next-generation technologies, the advancements in quantum clocks are a testament to the power of precision.
The future of measurement lies in harnessing the quantum realm, where even the smallest particles hold the key to groundbreaking discoveries.
Note: Materials provided above by The Brighter Side of News. Content may be edited for style and length.
Like these kind of feel good stories? Get The Brighter Side of News' newsletter.
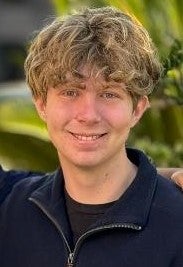