New quantum breakthrough could transform computing and communication
Researchers have unlocked ultrastrong photon-electron interactions, paving the way for next-gen quantum computing and communication.
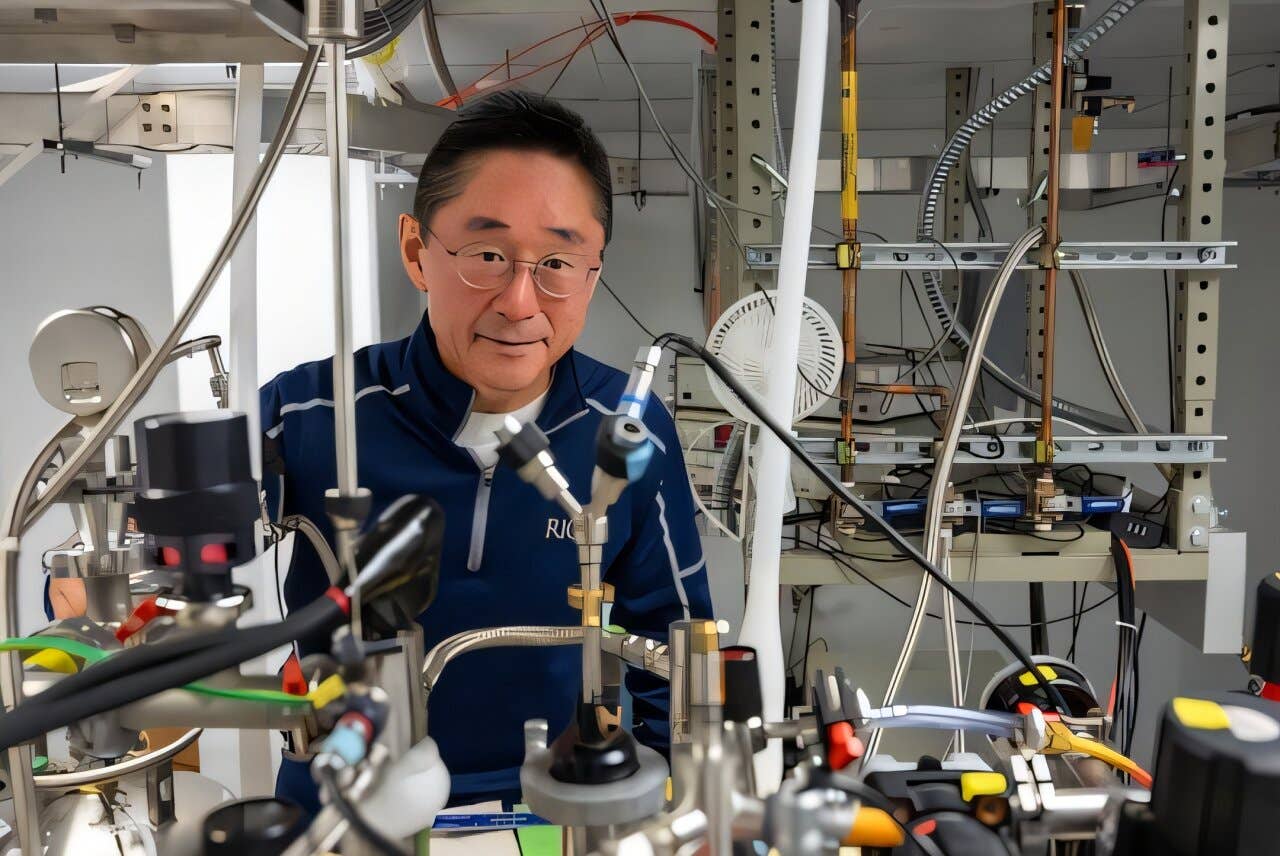
Junichiro Kono, a senior author of the study and professor at Rice University. (CREDIT: George Vidal / Rice University)
Quantum computing promises revolutionary advancements, capable of outperforming traditional technology in speed, accuracy, and security. Yet, the delicate quantum states required for such systems have long been challenging to sustain.
Researchers at Rice University have made a significant leap in overcoming these barriers by developing an advanced structure known as a 3D photonic-crystal cavity.
Their findings, published recently in Nature Communications, demonstrate innovative ways to manipulate light and matter interactions, paving the path for groundbreaking quantum technologies.
Controlling Light in New Ways
When photons—particles of light—interact with electrons, they form new quantum states called polaritons. These hybrid states blend properties of both particles, allowing scientists to control and manipulate light at incredibly tiny scales. Rice researchers constructed a sophisticated 3-dimensional optical cavity to explore these interactions, going beyond simpler 1-dimensional designs commonly used in prior studies.
Fuyang Tay, first author of the study and currently a postdoctoral researcher at Columbia University, compares their innovative cavity to a room filled with mirrors. “If you shine a flashlight inside, the light will bounce back and forth, reflecting endlessly. This is similar to how an optical cavity works—a tailored structure that traps light between reflective surfaces, allowing it to bounce around in specific patterns,” Tay said.
The specific patterns Tay describes are known as cavity modes, discrete frequencies where trapped photons resonate. These modes amplify the interaction between light and matter, crucial for quantum computing, precise lasers, advanced sensors, and faster fiber-optic communication networks.
Related Stories
Discovering Ultrastrong Coupling
A particularly intriguing outcome from this research is the observation of a phenomenon known as ultrastrong coupling. In simpler terms, this occurs when photons and electrons interact so intensely that their identities blend into entirely new states. Tay explains, “Ultrastrong coupling describes an unusual mode of interaction between light and matter where the two become deeply hybridized.”
Researchers achieved this unique regime by introducing electrons—confined to an ultrathin layer—into their 3D cavity under strong magnetic fields. They observed how electrons responded to different cavity modes simultaneously, especially when exposed to terahertz radiation, a type of electromagnetic wave. These conditions created ideal environments for ultrastrong coupling.
“Electrons strongly interact with each other, but photons typically don’t,” said Junichiro Kono, a senior author of the study and professor at Rice University. "This cavity confines light, strongly enhancing electromagnetic fields and leading to strong coupling between light and matter, creating quantum superposition states known as polaritons."
Photon-Photon Communication Through Matter
Another groundbreaking aspect of their work involved matter-mediated photon-photon coupling. Initially focused on enhancing interactions between light and matter, the team soon realized their setup allowed photons to communicate indirectly through electrons. This discovery sparked excitement among the researchers.
Andrey Baydin, assistant research professor at Rice, described the moment as an unexpected breakthrough. “This matter-mediated photon-photon coupling can lead to new protocols and algorithms in quantum computation and quantum communications,” added Kono.
By rotating the polarization—the orientation of the incoming light waves—the researchers observed two distinctly different behaviors. “Depending on the polarization of the light, the cavity modes either remain independent, or they mix together, forming completely new hybrid modes,” Tay explained. “This suggests we can engineer materials where different cavity modes ‘talk’ to each other through electrons in a magnetic field, creating new correlated states.”
Pushing Beyond Previous Limitations
Previous studies primarily used simpler 1-dimensional cavities—essentially stacked layers of reflective materials known as Bragg mirrors. While these are effective, their simplicity limits spatial control over the trapped photons. A three-dimensional cavity structure overcomes this constraint, allowing researchers to observe novel interactions due to varying electromagnetic fields inside the cavity itself.
Three-dimensional cavities are significantly harder to build, yet essential for exploring complex quantum phenomena like ultrastrong coupling. Such cavities allow photons to interact with electrons in multiple directions, which can enhance quantum effects such as superradiant phase transitions—a phenomenon impossible with simpler uniform cavities.
The Rice team used an ultrahigh-quality electron gas made from gallium arsenide (GaAs), an advanced semiconductor material. The electrons in this material could move freely, enabling precise measurements and observations of interactions between electrons and photons within the cavity.
Combining Experiments with Simulations
To fully understand their findings, the researchers combined experimental data with advanced computer simulations. Alessandro Alabastri, an assistant professor at Rice University, along with postdoctoral researcher Stephen Sanders, developed detailed computational models of their photonic structure. These simulations accurately reproduced their experimental observations, confirming the researchers’ interpretation of the complex quantum interactions.
Alabastri particularly appreciated Tay’s willingness to explore computational methods alongside laboratory experiments. “He is an experimentalist, but what I found really interesting is that he was willing to learn the computational part as well,” Alabastri said.
These simulations helped clarify how different cavity modes interact through electrons, offering insights essential for future quantum device designs. Researchers plan to leverage these findings to engineer new quantum materials, processors, and communication devices.
Future of Quantum Technologies
The research marks a significant step toward practical quantum technologies. By demonstrating robust methods to control quantum states, the Rice team has opened doors to advancements across multiple fields, from quantum computing to highly sensitive sensors.
Kono emphasized the importance of protecting delicate quantum states, saying, “Quantum phenomena or states are famously fragile. Cavity quantum electrodynamics is an emerging field where the cavity setting provides a controlled environment for protecting and harnessing quantum states.”
The findings highlight the powerful potential of three-dimensional photonic structures to revolutionize quantum science. Though significant challenges remain, this research demonstrates the feasibility of previously theoretical quantum phenomena, bringing scientists closer to building practical quantum computers and communication networks.
As Tay and his colleagues continue refining their approach, their work lays critical groundwork for transformative quantum technologies that could redefine computing and communication worldwide.
Note: The article above provided above by The Brighter Side of News.
Like these kind of feel good stories? Get The Brighter Side of News' newsletter.
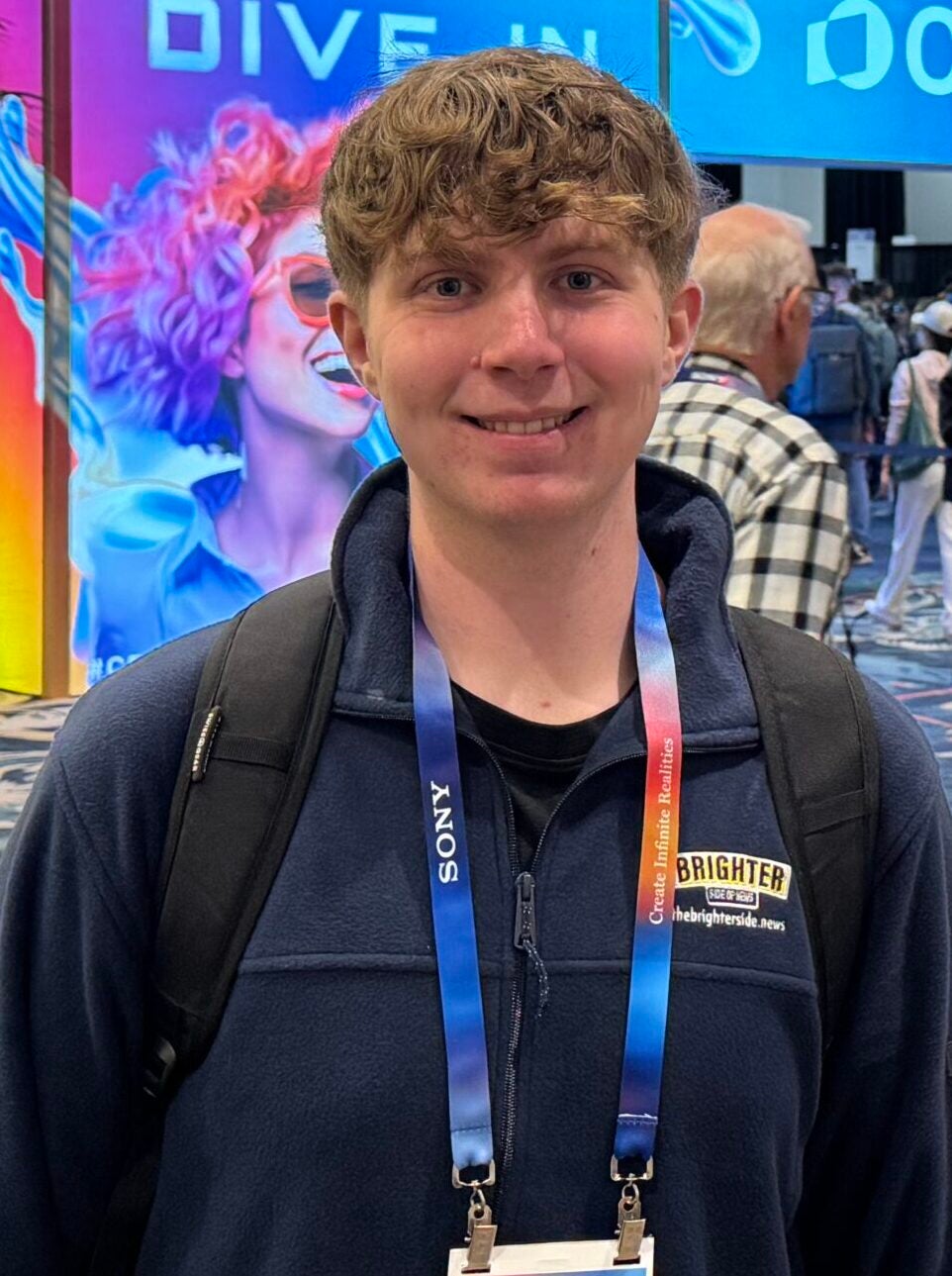
Joshua Shavit
Science & Technology Writer | AI and Robotics Reporter
Joshua Shavit is a Los Angeles-based science and technology writer with a passion for exploring the breakthroughs shaping the future. As a contributor to The Brighter Side of News, he focuses on positive and transformative advancements in AI, technology, physics, engineering, robotics and space science. Joshua is currently working towards a Bachelor of Science in Business Administration at the University of California, Berkeley. He combines his academic background with a talent for storytelling, making complex scientific discoveries engaging and accessible. His work highlights the innovators behind the ideas, bringing readers closer to the people driving progress.