New nanoscale transistors use quantum tunneling to achieve high performance and efficiency
MIT’s quantum tunneling transistors achieve high performance and energy efficiency, surpassing silicon’s limits with groundbreaking nanoengineering.
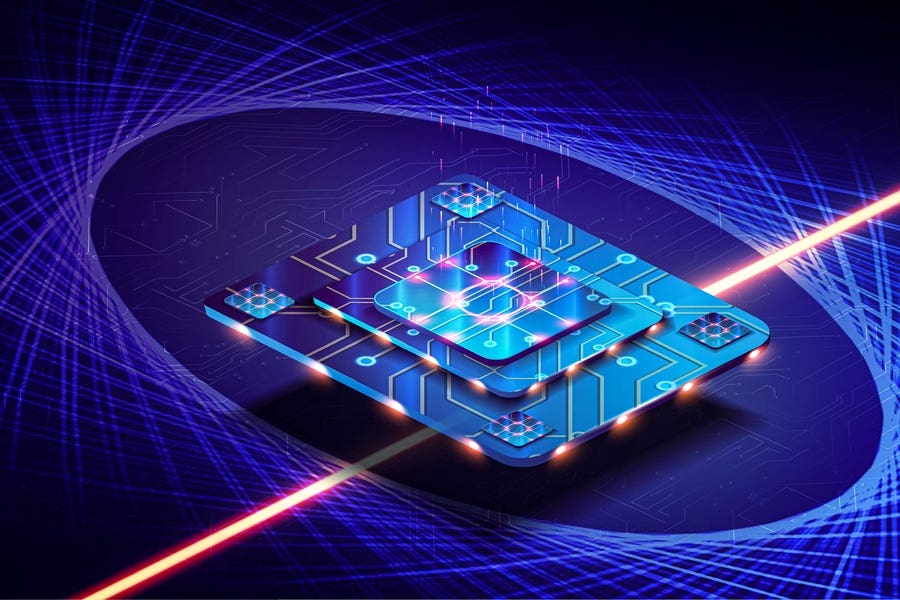
MIT researchers have developed vertical nanowire transistors using quantum tunneling, delivering unprecedented energy efficiency and performance. (CREDIT: CC BY-SA 4.0)
As computing demands grow, driven by artificial intelligence and the internet of things, the need for energy-efficient electronics becomes critical. At the heart of this evolution are transistors, the fundamental building blocks of modern devices. However, silicon-based transistors face fundamental limitations, known as the "Boltzmann tyranny," which restrict their efficiency and scalability.
Silicon transistors function by switching between "off" and "on" states when voltage is applied. This process requires a specific minimum voltage to work effectively, limiting energy efficiency.
Researchers at MIT have developed a revolutionary alternative—three-dimensional transistors leveraging quantum tunneling. These advanced devices promise to break silicon's physical barriers and usher in a new era of compact, energy-efficient computing.
Traditional silicon transistors operate with a subthreshold swing, a measure of voltage efficiency, limited to 60 mV per decade at room temperature. This constraint arises from fundamental electron behavior and hampers efforts to reduce power consumption further. The steepness of a transistor's switching slope reflects its energy efficiency; the sharper the slope, the lower the voltage needed.
By using gallium antimonide and indium arsenide instead of silicon, MIT researchers created vertical nanowire transistors. Published in the journal, Nature Electronics, these devices exploit quantum tunneling—a phenomenon where electrons penetrate energy barriers instead of moving over them. This design enables sharp switching slopes and efficient low-voltage operation.
"With conventional physics, there is only so far you can go," said Professor Jesús del Alamo, a senior author of the study. "This work shows we can do better by leveraging different physics."
In addition to quantum tunneling, these transistors harness extreme quantum confinement, where electrons are restricted to spaces so small that their behavior changes. This effect enables stronger tunneling currents and more efficient energy transfer. By focusing on heterojunction designs and employing precise engineering, the researchers achieved breakthroughs that traditional silicon technology cannot match.
Quantum confinement, a phenomenon where electrons are restricted to extremely small spaces, plays a pivotal role in this breakthrough. When confined, electron behavior changes, enhancing their ability to tunnel through barriers. MIT’s vertical nanowire transistors, with diameters as small as six nanometers, harness this effect to achieve unprecedented performance.
The precise engineering required for such devices presented significant challenges. The team’s use of MIT.nano’s state-of-the-art facilities allowed them to fabricate the smallest 3D transistors reported to date. This innovation enabled the devices to achieve both sharp switching slopes and high current density simultaneously—a feat not previously accomplished.
Related Stories
"We have a lot of flexibility to design these material heterostructures," said Yanjie Shao, lead author and postdoctoral researcher. "This allows us to achieve very thin tunneling barriers and high current densities."
MIT’s approach focused on vertical configurations of nanowire transistors. Unlike planar designs, vertical structures allow better control over short-channel effects, essential for maintaining performance as devices shrink. Moreover, the gate-all-around geometry ensures tight electrostatic control, maximizing efficiency even on a minuscule footprint.
The new transistors deliver a drive current of 300 µA per micrometer and operate at just 0.3 volts. Their switching slopes fall below the fundamental 60 mV per decade limit of silicon, achieving a peak transconductance of 1,050 µS per micrometer. This represents a twentyfold improvement over similar tunneling transistors.
The devices leverage interface-pinned band alignment and the quantum mechanical properties of gallium antimonide and indium arsenide. These materials form a broken-band heterojunction that facilitates efficient electron tunneling. This combination of advanced materials and precise design underpins the breakthrough performance metrics.
“This is the first time we have been able to achieve such sharp switching steepness with this design,” Shao said. Such advancements demonstrate the potential to surpass silicon in both performance and energy efficiency.
In addition to their impressive electrical characteristics, these transistors offer scalability unmatched by conventional silicon devices. By using vertical nanowires, the team decoupled gate length from footprint size, allowing flexible scaling without compromising performance. This capability positions these transistors as strong candidates for next-generation computing applications.
While these transistors show immense promise, significant work remains before they can be commercialized. The researchers aim to enhance fabrication methods to improve uniformity across entire chips.
Variations as small as one nanometer can impact electron behavior and device performance. Alternative designs, such as vertical fin-shaped structures, are also being explored to further optimize device uniformity.
"There are many challenges yet to be overcome for this approach to be commercial," del Alamo noted. "But conceptually, it really is a breakthrough."
Scaling challenges are not limited to uniformity. The integration of these transistors with existing silicon-based manufacturing processes presents hurdles. Compatibility with current fabrication infrastructure is essential for widespread adoption. To address these issues, the team is collaborating with industry experts to develop practical solutions.
The researchers are also investigating methods to further reduce energy consumption. By fine-tuning material properties and device geometries, they hope to push performance metrics even further. These advancements could enable applications in fields ranging from high-performance computing to energy-efficient wearable devices.
These innovations in nanoelectronics mark a pivotal step toward more energy-efficient computing. As artificial intelligence and data-driven technologies continue to expand, the demand for smaller, faster, and more efficient transistors will only grow.
By leveraging quantum mechanical properties, MIT’s vertical nanowire transistors pave the way for a future where powerful electronics operate with minimal energy consumption. This breakthrough has the potential to redefine the limits of technology, ensuring that computing can keep pace with the demands of tomorrow.
The potential applications of these transistors extend beyond computing. They could revolutionize sectors like telecommunications, automotive electronics, and renewable energy systems. Their ability to deliver high performance at low energy costs aligns with global efforts to reduce carbon footprints and enhance sustainability.
"This is a technology with the potential to replace silicon," Shao emphasized. "It offers the same functions but with much better energy efficiency."
The journey to commercialization will require overcoming technical and economic barriers, but the promise of this technology is undeniable. With continued research and innovation, these transistors could transform how devices are designed, manufactured, and utilized, setting new standards for efficiency and performance in the digital age.
Note: Materials provided above by The Brighter Side of News. Content may be edited for style and length.
Like these kind of feel good stories? Get The Brighter Side of News' newsletter.
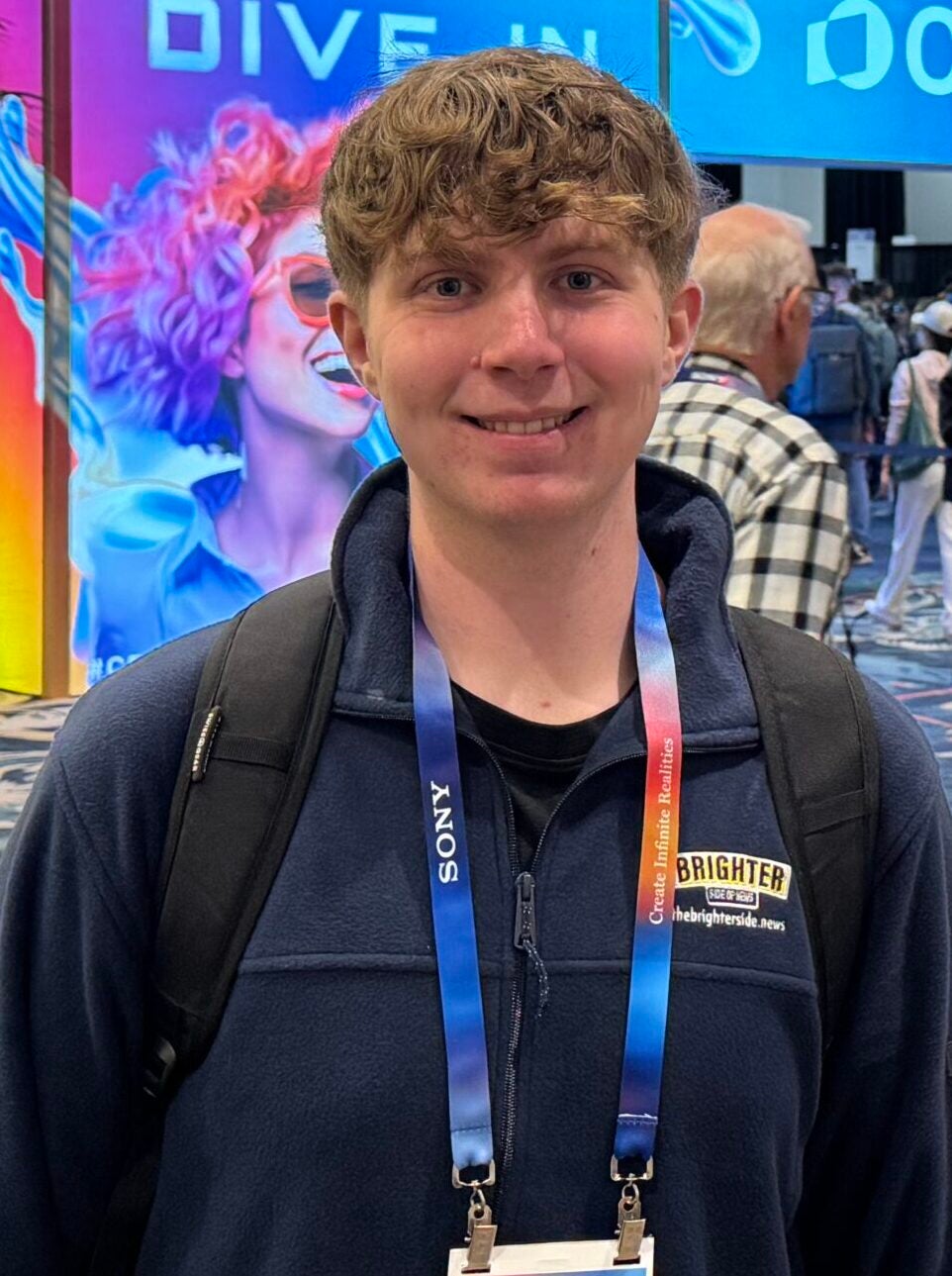
Joshua Shavit
Science & Technology Writer | AI and Robotics Reporter
Joshua Shavit is a Los Angeles-based science and technology writer with a passion for exploring the breakthroughs shaping the future. As a contributor to The Brighter Side of News, he focuses on positive and transformative advancements in AI, technology, physics, engineering, robotics and space science. Joshua is currently working towards a Bachelor of Science in Business Administration at the University of California, Berkeley. He combines his academic background with a talent for storytelling, making complex scientific discoveries engaging and accessible. His work highlights the innovators behind the ideas, bringing readers closer to the people driving progress.