Neutron ‘starquake’ discovery could revolutionize nuclear physics
Starquakes in neutron stars could test nuclear physics theories and advance nuclear energy, security, and medical imaging.
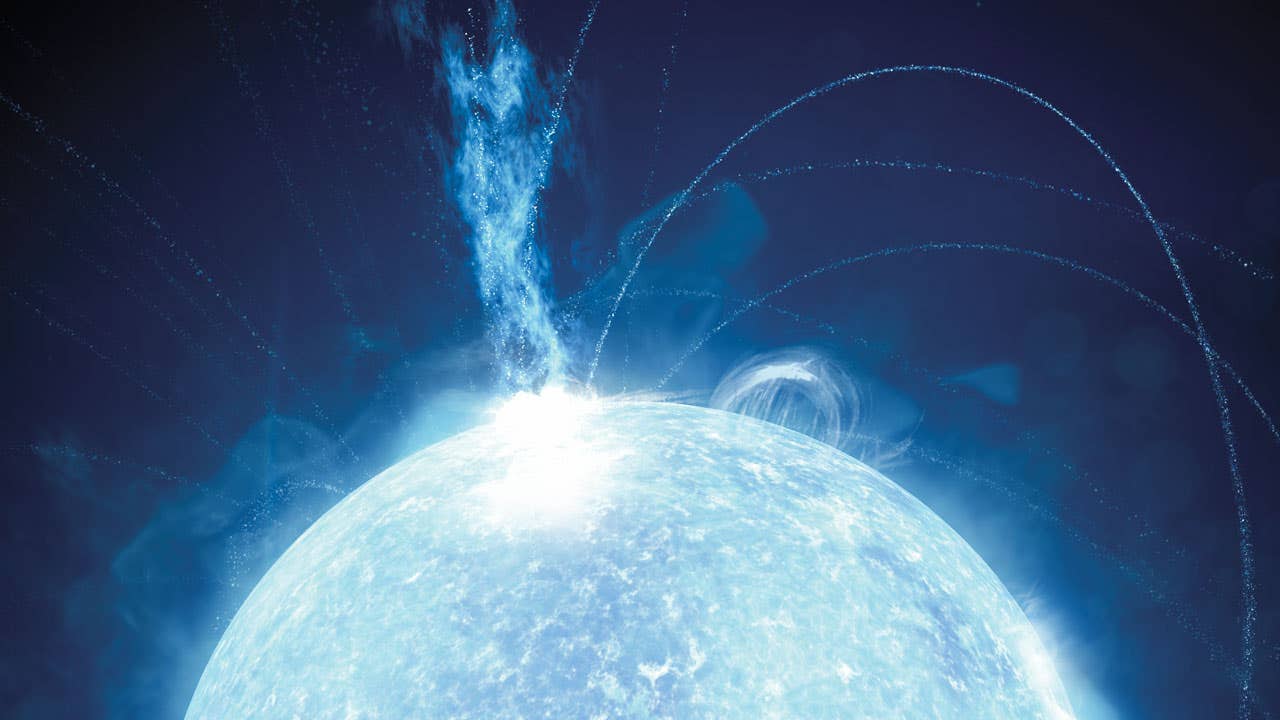
Neutron star quakes may unlock new insights into nuclear physics, challenging existing theories and advancing astronomy, energy, and medical imaging. (CREDIT: NASA’s Goddard Space Flight Center / Chris Smith / USRA / GESTAR)
Neutron stars, the collapsed remnants of massive stars, hold the densest matter in the universe. These cosmic objects are formed when a star runs out of fuel and collapses under its own gravity, leaving behind a dense core packed with protons and neutrons. Because their internal conditions are impossible to replicate on Earth, studying them could reveal fundamental insights into nuclear physics.
New research led by scientists at the University of Bath suggests that "starquakes"—vibrations and flares within neutron stars—could offer a powerful way to investigate the extreme nuclear matter inside them.
By analyzing these stellar tremors, physicists may be able to test a key nuclear theory called Chiral Effective Field Theory (χEFT), which describes how nuclear forces behave under extreme conditions.
“Our findings promise to add to, or change, the tools used by nuclear physicists, bringing astronomy and nuclear physics closer together,” said lead author Dr. Duncan Neill, a postdoctoral researcher at Bath. The study, published in Physical Review C, could reshape how scientists understand neutron stars, nuclear interactions, and even the universe itself.
Probing Nuclear Matter with Starquakes
At the core of neutron stars, nuclear matter is squeezed to densities far beyond what can be created in laboratories. Scientists rely on mathematical models like χEFT to predict how protons and neutrons interact under such conditions.
This theory, grounded in quantum chromodynamics (QCD), explains nuclear forces using an effective approach that accounts for the symmetries of low-energy QCD.
While χEFT has proven effective in describing atomic nuclei and neutron-rich matter at low densities, its accuracy at extreme densities remains uncertain. Testing it requires data from neutron stars, which naturally provide the conditions needed to evaluate the theory’s predictions.
Asteroseismology—the study of stellar vibrations—offers a novel way to investigate this. Neutron star quakes, triggered by shifts in the star’s crust or tidal interactions in binary systems, produce vibrations that travel through the star's interior.
Related Stories
These oscillations, known as normal modes, are influenced by the nuclear matter inside the star. By measuring these oscillations, scientists can infer details about neutron star structure and test χEFT predictions at high densities.
Starquakes and the Mystery of Nuclear Symmetry
One of the most important nuclear properties studied in neutron stars is the symmetry energy, which describes how the energy of nuclear matter changes with varying proton-to-neutron ratios.
While atomic nuclei on Earth contain nearly equal numbers of protons and neutrons, neutron stars are overwhelmingly neutron-rich. This means symmetry energy plays a crucial role in determining neutron star properties, including their structure and stability.
Laboratory experiments using heavy-ion collisions and nuclear binding energy measurements have constrained symmetry energy at densities near nuclear saturation (0.16 particles per cubic femtometer). However, neutron stars reach densities multiple times higher, where experimental data is scarce. Measuring starquakes provides a way to extend symmetry energy studies into this unexplored high-density regime.
Recent experiments such as the PREX-II and CREX projects have provided conflicting results on neutron-rich nuclei, highlighting the challenge of determining symmetry energy from laboratory data alone. Neutron stars offer an alternative approach:
By observing resonant shattering flares (RSFs)—brief gamma-ray bursts triggered when a neutron star's crust fractures due to tidal forces—scientists can measure oscillation frequencies linked to the star’s symmetry energy.
Dr. David Tsang, a co-author of the study, explained, “We propose that, in the near future, asteroseismology could be used to obtain granular detail about matter inside neutron stars, and thus test theories like Chiral Effective Field Theory.”
A New Era for Nuclear Physics and Astronomy
Observing neutron star vibrations could revolutionize the study of nuclear matter, linking astronomy and nuclear physics in unprecedented ways.
Current methods for probing neutron stars rely on indirect measurements such as X-ray emissions, gravitational waves, and relativistic effects like Shapiro delay. While these approaches have provided constraints on neutron star masses and radii, they offer limited insights into nuclear interactions at high densities.
Starquakes and RSFs, on the other hand, provide direct measurements of neutron star vibrations. The crust-core interface mode (i-mode), for example, is particularly sensitive to the nuclear matter equation of state at densities around half nuclear saturation.
Detecting and analyzing this mode could place new constraints on the fundamental properties of nuclear matter, potentially resolving discrepancies between nuclear models and astronomical observations.
The implications extend beyond astrophysics. A deeper understanding of nuclear forces could lead to advancements in:
- Medical technology: Improved models of nuclear interactions could enhance radiation therapy and diagnostic imaging.
- National security: Better nuclear physics knowledge is crucial for maintaining and monitoring nuclear stockpiles.
- Nuclear energy: Understanding nuclear matter at extreme conditions could inform the development of safer, more efficient nuclear reactors.
Dr. Neill emphasized the practical benefits of this research: “The asteroseismic techniques we propose have the advantage of using instruments already in operation, giving new applications to existing telescopes and expanding the tools of nuclear physics without requiring expensive new developments.”
As more neutron stars are observed using multi-messenger astronomy—combining gravitational wave data with electromagnetic observations—the potential for asteroseismology will only grow. Scientists hope to eventually map out the nuclear equation of state across different density ranges, offering a clearer picture of how nuclear matter behaves under extreme conditions.
“We hope to expand our research in asteroseismology at Bath, seeing just how much it could tell us,” Neill added.
By transforming starquakes into scientific tools, neutron stars could soon become nature’s most powerful nuclear laboratories, offering a window into the fundamental forces shaping the universe.
Note: Materials provided above by The Brighter Side of News. Content may be edited for style and length.
Like these kind of feel good stories? Get The Brighter Side of News' newsletter.
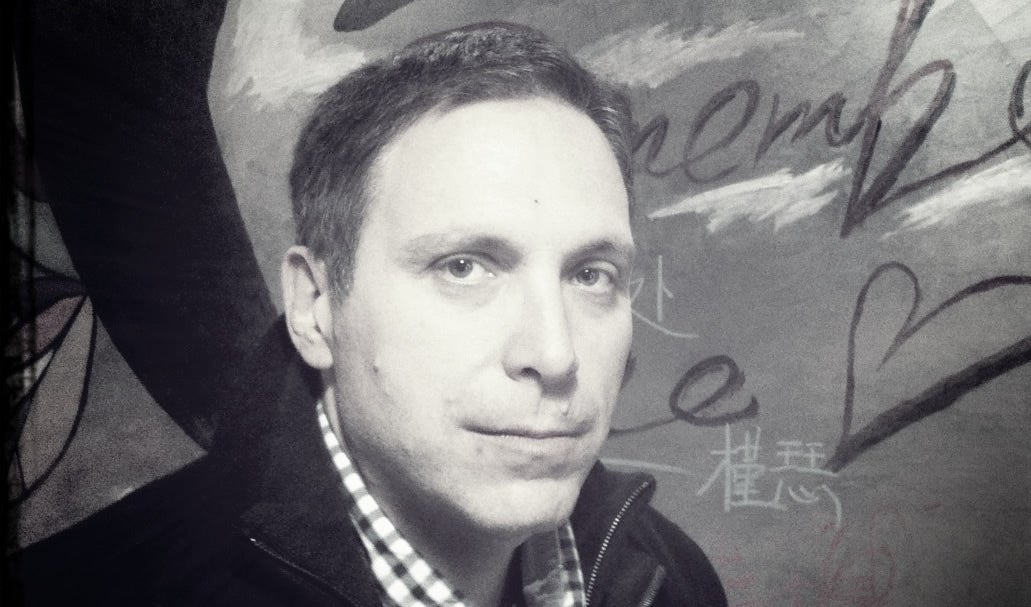
Joseph Shavit
Head Science News Writer | Communicating Innovation & Discovery
Based in Los Angeles, Joseph Shavit is an accomplished science journalist, head science news writer and co-founder at The Brighter Side of News, where he translates cutting-edge discoveries into compelling stories for a broad audience. With a strong background spanning science, business, product management, media leadership, and entrepreneurship, Joseph brings a unique perspective to science communication. His expertise allows him to uncover the intersection of technological advancements and market potential, shedding light on how groundbreaking research evolves into transformative products and industries.