MIT engineers’ revolutionize the design and operation of wind farms
MIT engineers have developed a groundbreaking model to optimize wind turbine performance and wind farm operations, marking a major advance in renewable energy technology.
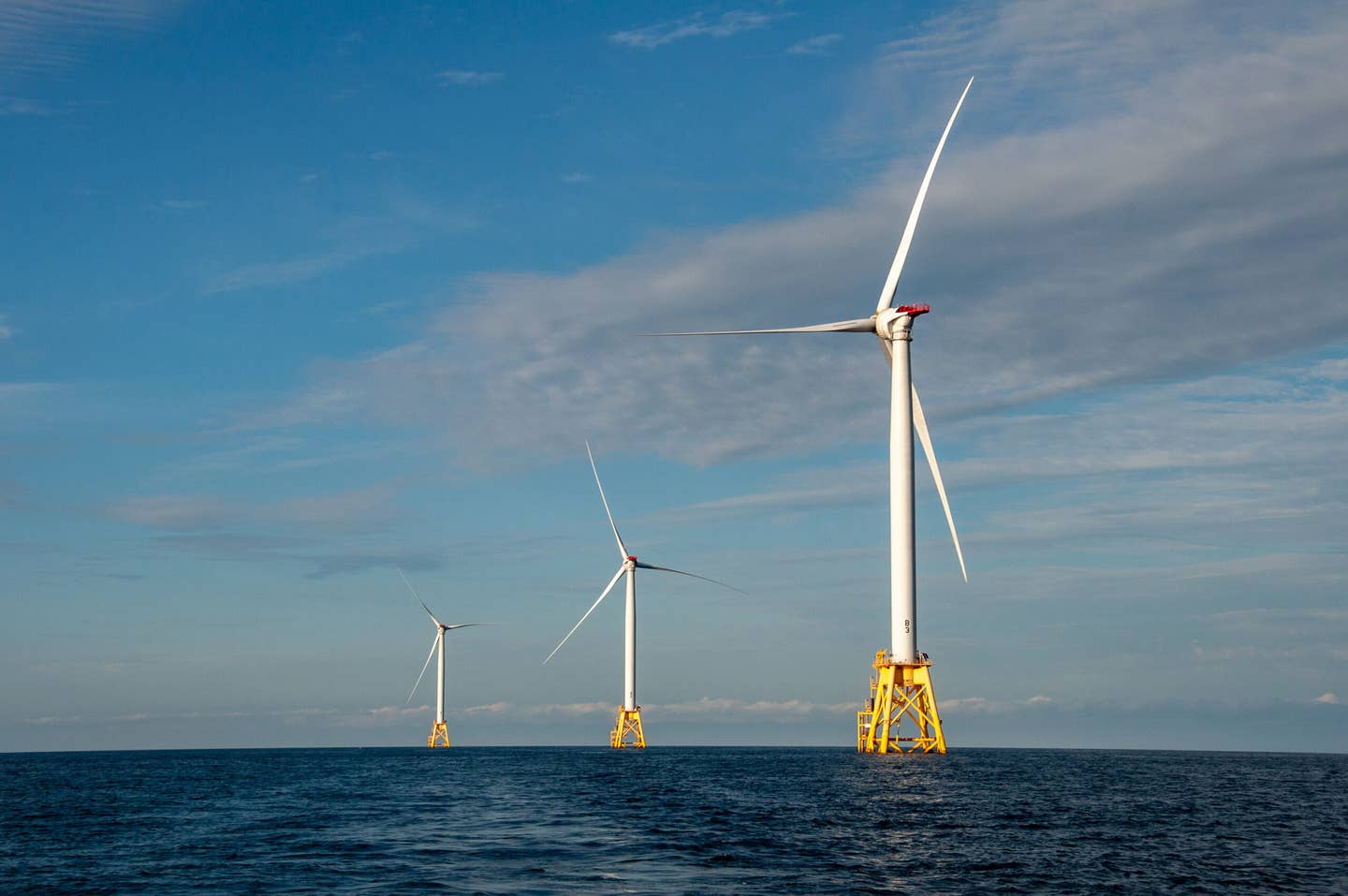
A team of engineers at MIT has now developed a comprehensive physics-based model that accurately represents the airflow around rotors, even under extreme conditions. (CREDIT: CC BY-SA 3.0)
Engineers have long known that traditional aerodynamics models, used to design the blades of wind turbines and propellers, don’t account for certain real-world conditions. Though these formulas, established over a century ago, work well in basic scenarios, they falter when applied to high forces, extreme speeds, or complex angles.
This has led engineers to rely on “correction factors,” essentially patching up the gaps with empirical data and trial and error. However, this workaround has always been less than ideal.
A team of engineers at MIT has now developed a comprehensive physics-based model that accurately represents the airflow around rotors, even under extreme conditions. This new approach could revolutionize the design of rotors and the layout and operation of wind farms.
The research, published in Nature Communications, was conducted by MIT postdoc Jaime Liew, doctoral student Kirby Heck, and Assistant Professor Michael Howland.
“We’ve developed a new theory for the aerodynamics of rotors,” says Howland. This new model can calculate the forces, flow velocities, and power of a rotor, whether it’s extracting energy from airflow, like a wind turbine, or adding energy to the flow, like a ship’s propeller. “The theory works in both directions,” Howland adds.
Optimizing Wind Farms
One of the most immediate applications of this model lies in wind farm operation. Operators need to constantly adjust variables such as the orientation of turbines, rotation speeds, and blade angles to optimize power generation while keeping things safe. Currently, these adjustments are often based on guesswork and experience, but the new model offers a fast, data-driven approach to optimization.
Related Stories
Howland expresses excitement about this breakthrough, saying, “It has immediate and direct potential for impact across the value chain of wind power.”
This marks a significant departure from the previous modeling approach, known as momentum theory. Developed in the 19th century, momentum theory allowed engineers to predict how much power a rotor could generate or how much energy would be needed to propel a ship or plane.
The theory was widely adopted and remains a staple in wind energy textbooks. It even led physicist Albert Betz to calculate what is now known as the Betz limit—the maximum amount of energy, 59.3%, that can theoretically be extracted from the wind.
However, the limitations of momentum theory became apparent as engineers pushed the boundaries of what rotors could do. When blades rotate at higher speeds or operate at different angles, the theory not only miscalculates the amount of force but also the direction.
According to Howland, experiments have shown that at higher speeds or sharper blade angles, the force actually continues to increase, contradicting the theory’s predictions. “It’s not just quantitatively wrong, it’s qualitatively wrong,” Howland points out.
New Insights for a Century-Old Challenge
One of the most significant shortcomings of the older theory is its inability to handle misaligned turbines—an issue that’s quite common in wind farms, where wind direction constantly shifts. In a 2022 paper, Howland and his team found that deliberately misaligning some turbines in a wind farm can actually boost overall power output. This is because slight misalignments can reduce wake disturbances, allowing downstream turbines to generate more energy.
Up until now, engineers have addressed these issues with ad hoc fixes, tweaking original formulas based on wind tunnel tests and experience in the field. But there’s been no solid theoretical framework for these adjustments—until now.
Howland’s team tackled the problem by creating detailed computational models of rotor aerodynamics. One key finding was that the original model assumed that air pressure behind the rotor would quickly return to normal, but this assumption turned out to be flawed. As thrust force increases, the pressure drop lingers much longer than the old model predicted.
This discrepancy becomes particularly problematic near the Betz limit—the point where turbines are expected to perform at their best. Howland explains, “We have Betz’s prediction of where we should operate turbines, and within 10 percent of that operational set point, the theory completely deteriorates.”
The team’s new unified momentum model fixes these issues by incorporating three-dimensional aerodynamics principles, typically used in aerospace engineering. They tested and validated this model using computational fluid dynamics, and further validation is underway through wind tunnel and field tests.
A New Take on the Betz Limit
Interestingly, the new model has led to a revision of the Betz limit itself. While the change is small—just a few percentage points—it's a notable update to a rule of thumb that’s stood for over 100 years. “Now we have a new theory, and the Betz limit that’s been the rule of thumb for a hundred years is actually modified,” says Howland. “And that’s immediately useful.”
The new model allows for improved power generation even when turbines are misaligned with airflow, something the Betz limit couldn’t account for.
What’s more, the model’s benefits can be applied immediately, without any need for changes to the hardware of existing wind farms. In fact, the new model builds on earlier work by Howland’s team, which demonstrated that adjusting turbines’ alignment could increase overall efficiency.
“This breakthrough is a natural extension of our previous work on optimizing utility-scale wind farms,” Howland says. The older methods of predicting power output were based on empirical data, which, while useful, lacked the precision needed to fully optimize turbine performance.
Wake modeling—the process of understanding how the wake of one turbine affects others—is crucial for both wind farm design and day-to-day operation. As turbines extract energy from the wind, they create a wake that reduces the wind’s energy for neighboring turbines. Being able to predict and control these wake effects can significantly enhance a wind farm’s efficiency.
Until now, wind farm operators, turbine manufacturers, and blade designers had no reliable way to predict how changes like blade angle or rotation speed would affect power output without relying on empirical corrections. “That’s because there was no theory for it,” Howland explains. “Our theory can directly tell you, without any empirical corrections, for the first time, how you should actually operate a wind turbine to maximize its power.”
Broader Applications
While the research focuses on wind turbines, the new model applies to any rotor-based system, including propellers for airplanes and ships, as well as hydrokinetic turbines used in tidal or river energy generation. The model’s broad applicability stems from its focus on fluid dynamics principles, which are similar across air and water.
Though Howland’s team didn’t focus on these other applications in this study, the model’s theoretical underpinnings naturally extend to them.
The new theory is available in the form of mathematical formulas, which can be incorporated into software for rapid prototyping, control, and optimization. The team has also made an open-source version available on GitHub, making it easy for engineers and researchers to start using it immediately.
“We developed this model for fast-running tools, so it can be used for rapid prototyping and real-time control,” Howland says. The goal is to enable faster progress in wind energy research and to help boost wind power capacity and reliability—key factors in the fight against climate change.
Note: Materials provided above by The Brighter Side of News. Content may be edited for style and length.
Like these kind of feel good stories? Get The Brighter Side of News' newsletter.
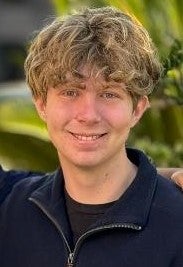