MIT breakthrough: Turning CO2 into useful products just got easier
Researchers develop a scalable electrode design for efficient CO2 conversion, paving the way for sustainable production of ethylene and other chemicals.
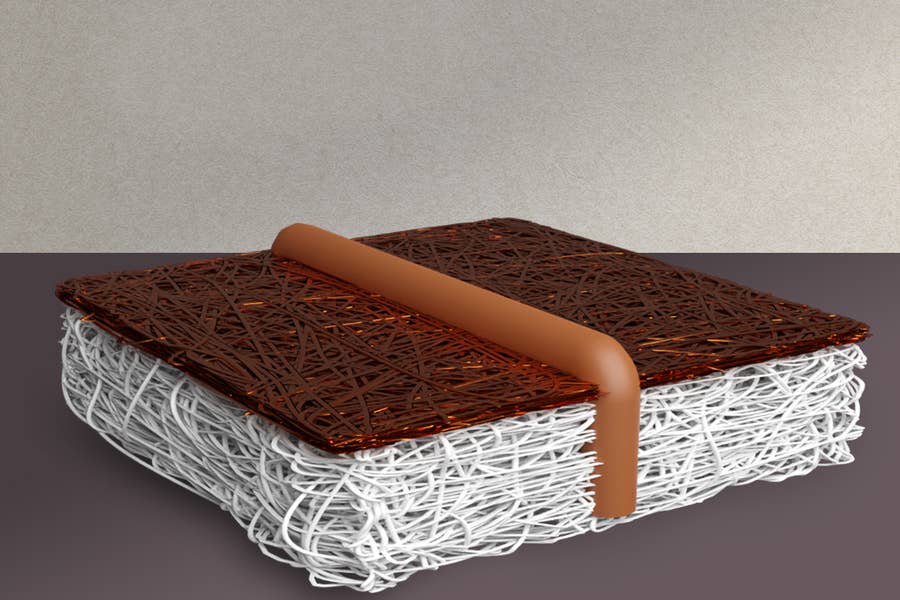
A conceptual schematic of the new woven electrode design. Researchers wove a series of conductive copper wires (the brown-orange pipe) through a very thin membrane to reach the catalyst. (CREDIT: MIT)
As the world battles to reduce greenhouse gas emissions, researchers are turning to innovative ways to repurpose carbon dioxide.
One promising approach is electrochemical CO2 reduction, which converts captured CO2 into valuable products like fuels, chemicals, and building materials. When powered by renewable electricity, this process offers dual benefits: energy storage and net-negative carbon sequestration.
In recent years, advances in catalysts, reaction pathways, and electrode designs have brought this technology closer to commercial viability. Laboratory tests using small electrodes have achieved promising results. These include carbon dioxide utilization rates of up to 90% and electrical efficiencies nearing 45%. Yet, the challenge lies in scaling these systems while maintaining performance.
The electrochemical reduction of CO2 to ethylene—a key ingredient in plastics and fuels—is of particular interest. Currently, ethylene is produced from petroleum and sells for approximately $1,000 per ton. Meeting or beating this cost using CO2 could revolutionize the industry.
Addressing the Scale Problem
At the heart of this technology are gas diffusion electrodes, which drive the conversion process. These electrodes face a critical design trade-off. They must conduct electricity efficiently while repelling water to prevent interference with the chemical reaction. Enhancing conductivity often diminishes water-repelling properties, and vice versa.
Engineers at MIT have now developed a solution that tackles this conflict head-on. By embedding conductive copper wires into PTFE—a hydrophobic plastic—researchers created a material that excels in both conductivity and water resistance. This breakthrough, led by MIT doctoral student Simon Rufer and Professor Kripa Varanasi, is detailed in Nature Communications.
“This work really addressed this challenge,” says Varanasi. “We can now get both conductivity and hydrophobicity.”
A Simple Yet Powerful Innovation
The team’s approach involved weaving copper wires through thin PTFE sheets. Copper acts as a "superhighway" for electrons, minimizing resistance. This weaving technique effectively divides the material into smaller, high-performing sub-electrodes.
Related Stories
The innovation also enables larger electrodes, essential for industrial applications. While most lab tests use electrodes around 2.5 centimeters in size, the MIT team successfully scaled their design to 10 times that area without losing efficiency.
“It’s the first PTFE-based electrode to progress beyond the lab scale without sacrificing efficiency,” Rufer explains. The system maintained stable performance over 75 hours of continuous testing, demonstrating its robustness.
To support larger-scale applications, the team developed a model to optimize wire spacing, ensuring even distribution of electrical conductivity. This innovation addresses a major bottleneck: as electrode size increases, energy requirements typically surge, making the process costlier.
Industrial applications will demand electrodes up to 100 times larger than current lab models. The researchers believe their woven-wire system can meet these demands. Moreover, the manufacturing process can be easily integrated into existing roll-to-roll systems, streamlining production.
Beyond Ethylene
While the study focused on ethylene, the technique is versatile. “You can sew this micrometric copper wire into any gas diffusion electrode, independent of catalyst morphology or chemistry,” Rufer says. This adaptability could benefit other high-value chemical products, including methane, methanol, and carbon monoxide.
Technoeconomic analyses suggest that scaling these lab results could make CO2-derived ethylene competitive with traditional methods. Additionally, carbon monoxide production is already nearing profitability under current lab conditions.
As Varanasi notes, scaling solutions to address gigaton-level CO2 emissions will be crucial in mitigating climate change. “Our hierarchically conductive electrode is a result of such thinking,” he says.
The research team, which includes MIT graduate students Michael Nitzsche, Sanjay Garimella, and Jack Lake, credits its success to a collaborative effort supported by Shell and the MIT Energy Initiative.
This innovation marks a significant step toward sustainable CO2 utilization, promising a future where captured carbon becomes a resource rather than a liability.
Note: Materials provided above by The Brighter Side of News. Content may be edited for style and length.
Like these kind of feel good stories? Get The Brighter Side of News' newsletter.