Microscopic robots are transforming health, technology, and the environment
Penn State researchers unlock how active particles move in tight spaces, paving the way for new microscale medical and material innovations.
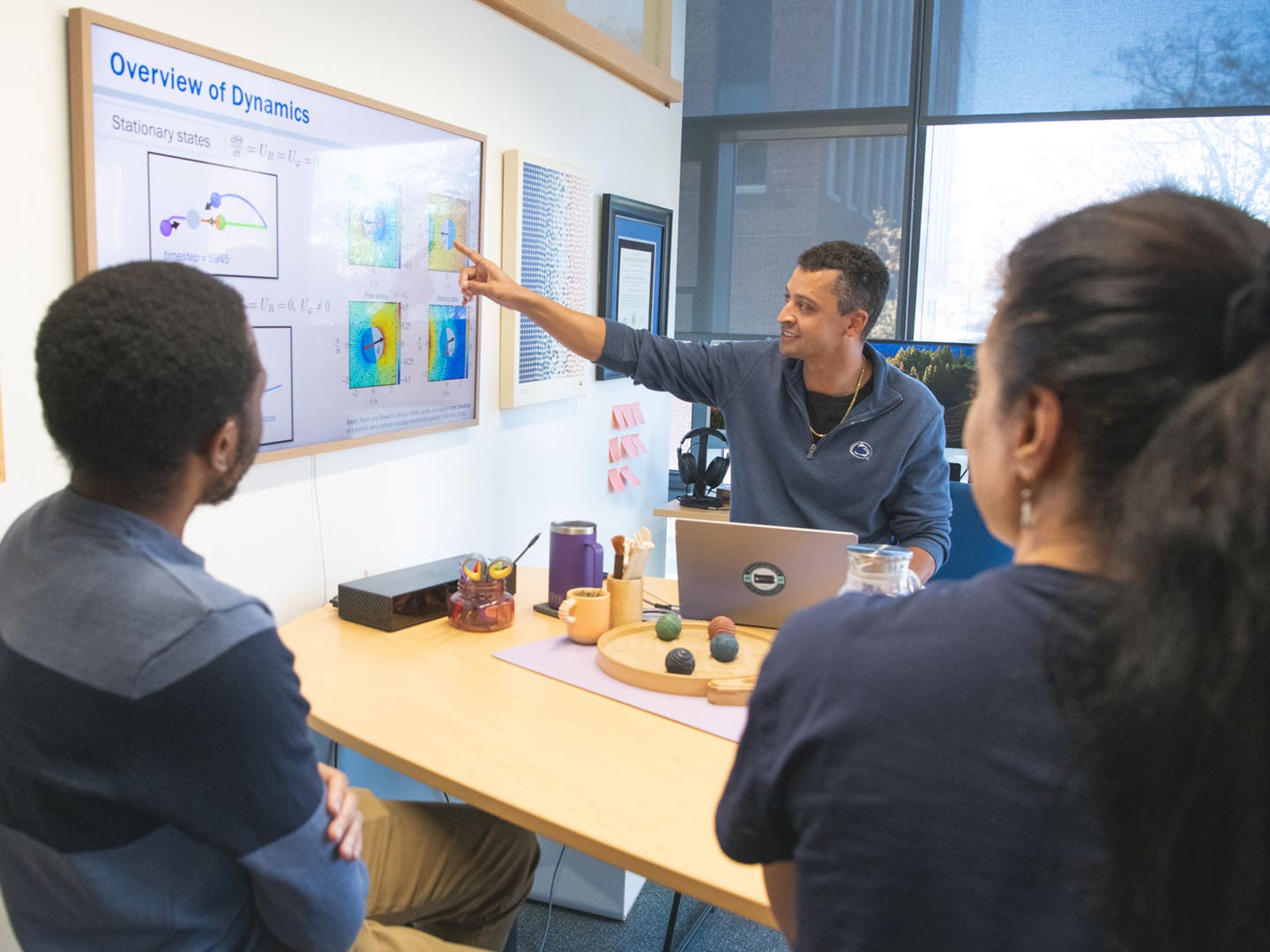
Stewart Mallory, assistant professor of chemistry and chemical engineering at Penn State, leads a research group that studies active matter, specifically the collective behavior of self-propelled microscopic particles. (CREDIT: Michelle Bixby / Penn State)
Tiny particles are opening big doors for medicine, materials science, and even environmental cleanup. Across the world, scientists are racing to understand how these microscopic swimmers behave, interact, and can be controlled to solve some of today's largest challenges. At the forefront of this work is Stewart Mallory, an assistant professor at Penn State, who is tackling some of the most stubborn mysteries of active matter.
Active matter, a field studying systems composed of self-propelled particles, holds incredible promise. Whether it is microscopic robots designed to deliver drugs inside your bloodstream or particles breaking down pollutants in oceans, these tiny machines depend on mastering how they move—especially when they face tight spaces.
Mallory’s team recently published a study in The Journal of Chemical Physics offering a new solution to a very old physics problem. How does a particle’s movement change when it’s squeezed into a narrow space where it cannot pass its neighbors? This classic situation, called single-file diffusion (SFD), turns out to have surprising importance for the future of micromachines.
Unlocking the Secrets of Single-File Diffusion
When you are stuck in traffic, unable to pass the car ahead, you are experiencing a version of SFD. Microscopic particles, when trapped in narrow channels, face a similar challenge. They can only move forward or backward, but never overtake. As a result, their movement becomes slow and unpredictable over long periods.
To crack this behavior, Mallory’s team used Brownian dynamics simulations, a way of modeling random motion at tiny scales. Building on earlier research by Schiltz-Rouse and others published in Physical Review E, they explored how a special kind of particle, known as an athermal active Brownian particle (ABP), moves under these tight conditions.
Their findings showed that at first, the movement looks "ballistic," meaning it moves fast and straight, influenced by the particle’s "kinetic temperature." This temperature is not from heat, but from the particle’s self-driven activity. Over time, though, the motion slows down and follows the typical SFD behavior, where the mean square displacement (MSD)—how far a particle has traveled—grows with the square root of time.
Related Stories
However, Mallory’s team uncovered something new. When particles propel themselves, it changes how easily they can move even in a one-dimensional line. They found a direct link between the mobility of these active particles and the compressibility of the system—the ability of particles to pack more tightly together under pressure.
The team even extended the 1D-mobility ideas originally proposed by Kollmann in 2003 to cover these active systems, a breakthrough that could help design better microscale devices.
"Before we can deploy these systems," Mallory explained, "we need to first run simulations to understand how these microscopic swimmers behave in complex environments."
This advancement is key for real-world applications, like guiding micro-robots through your blood vessels. By knowing exactly how long it would take a drug-delivering particle to reach its target, scientists can design better therapies that are faster and more reliable.
A Microscopic View of Human Behavior
Surprisingly, studying particles at the microscale changed how Mallory sees the world at large. Driving in traffic, for example, looks very different to him now. He points to "phantom traffic jams," those sudden slowdowns without any visible cause, as an example of single-file dynamics in daily life. Small changes in speed or distance between cars can build up into major jams, just as tiny fluctuations among active particles can cause them to clump together and slow down.
In both cases, whether it’s a nanoparticle or a car, the inability to pass neighbors and the need to respond to small disturbances in motion shape the entire system's behavior. "Our work on active particles moving in narrow channels has made me think a lot more about traffic," Mallory said.
This connection between micro and macro worlds underscores the broad relevance of active matter research. Lessons learned from the behavior of tiny swimmers can ripple outward, offering insights into everything from transportation to crowd control.
Tuning the Movement of Microswimmers
Mallory's team has not limited its focus to single-file diffusion. Earlier, they tackled another major challenge: how to control the movement of Phoretic Janus particles, another type of self-propelled nanoparticle.
These particles, first invented at Penn State about 20 years ago, have two chemically different sides. Like a tiny submarine with distinct front and back ends, they create chemical gradients that push them forward through a liquid. This unique structure earned them the name "Janus," after the Roman god of transitions. Before tackling this single file problem, Mallory's team published a paper in ACS Nano showing a potential way to tune Phoretic Janus particles.
By adjusting the surface chemistry of these particles, researchers can guide how they move in response to specific chemical signals. It’s a bit like steering a tiny car by choosing the right kind of fuel and tweaking its engine.
Mallory’s group has been working to understand how the fuel source—whether it’s hydrogen peroxide for metallic Janus particles or glucose for enzyme-coated ones—affects their speed and direction. Just as important, they are studying how these swimmers behave when many of them interact in the same space.
"On the individual level, we are aiming to control and accurately simulate the behavior of single Janus particles," Mallory said. "On the collective level, we explore how behavior changes when many particles interact."
These insights are crucial because real-world environments, whether inside a human body or a polluted river, involve many particles bumping into each other and influencing one another's paths.
Building New Materials and Healing the Environment
One of the most exciting frontiers for active matter lies in materials science and environmental cleanup. Mallory’s research shows that self-propelled particles could be used for self-assembly—the natural process where simple parts come together to form complex structures.
Nature uses self-assembly to build everything from virus shells to cell membranes. Scientists hope to harness this same process to create new materials by designing nanoparticles that can move and arrange themselves into desired patterns without external guidance.
"In our work," Mallory explained, "self-propelled particles can enhance this process, making self-assembly a more effective tool for building at the microscale."
Beyond building materials, active matter could help heal the planet. Some nanoparticles are designed to seek out and bind to microplastics, breaking them down into harmless substances. Others can detect specific chemical signals, like the acidic conditions created by cancer cells, and swim toward those cells to deliver targeted treatments.
"There are nanoparticles made of calcium carbonate that respond to pH gradients generated by cancer cells," Mallory said. "At some point, we could use those particles to carry a payload of medication and target harmful cells like cancer."
The possibilities extend beyond medicine to cleaning oceans and rivers, offering hope for tackling some of the toughest environmental challenges.
Moving Forward: Simulating the Future
Today, Mallory’s lab is focused on building better theories and computational models to predict particle behavior in all kinds of environments. These models are essential for designing microscale devices that must navigate complex and changing conditions.
The group’s work contributes to a growing body of knowledge that will eventually allow scientists to create smarter, more adaptable particles. Their findings are already influencing research across chemistry, physics, and engineering, helping others imagine new ways to use active matter for the good of society.
"Any step we make is a step forward in understanding and manipulating matter at the microscale," Mallory said.
As science moves deeper into the tiny world of active matter, the results could transform how materials are made, how diseases are treated, and how the environment is restored. From a jammed-up particle in a microscopic channel to a tiny robot delivering medicine with pinpoint accuracy, the power of the small may soon change the very fabric of your world.
Note: The article above provided above by The Brighter Side of News.
Like these kind of feel good stories? Get The Brighter Side of News' newsletter.
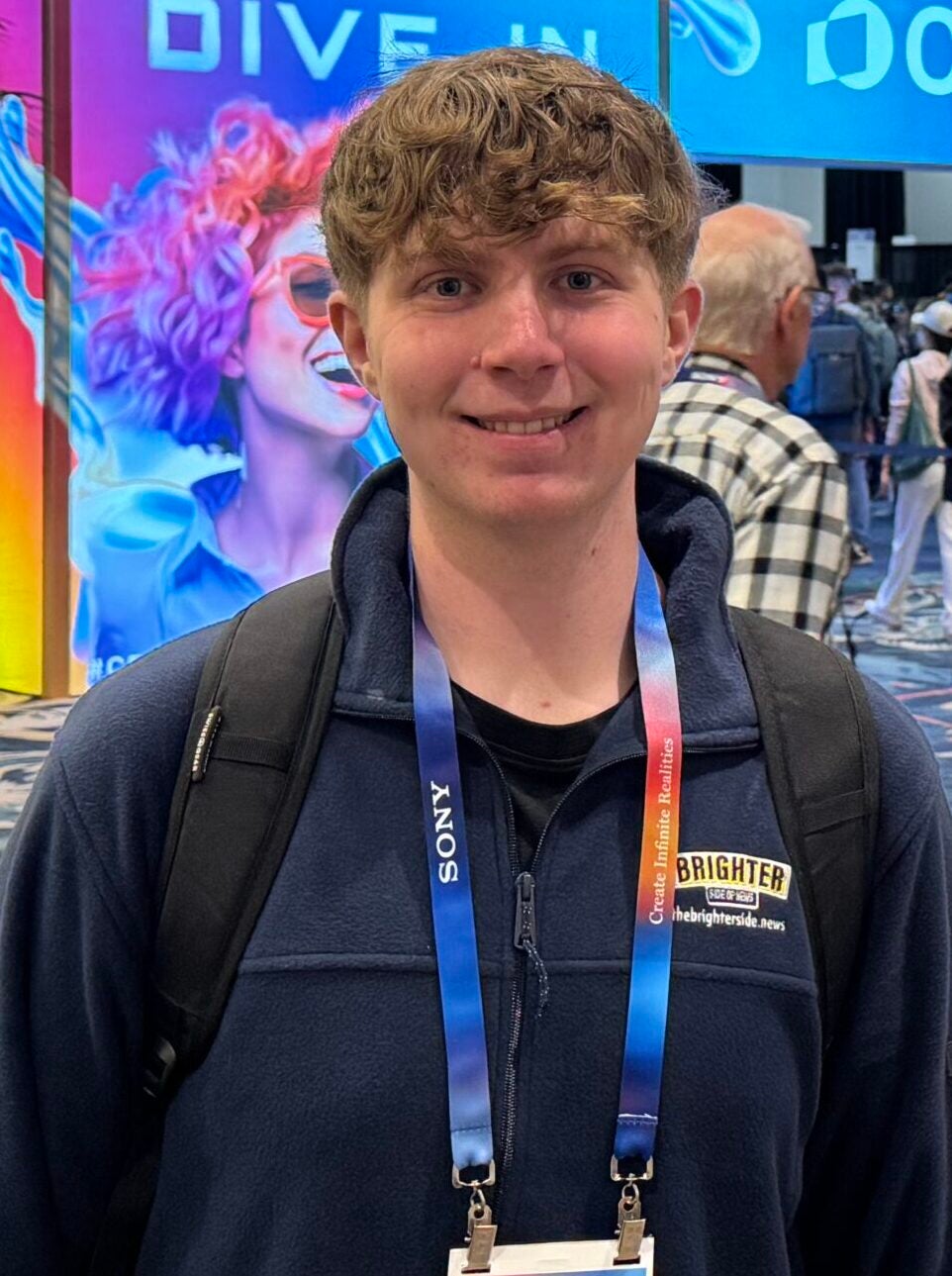
Joshua Shavit
Science & Technology Writer | AI and Robotics Reporter
Joshua Shavit is a Los Angeles-based science and technology writer with a passion for exploring the breakthroughs shaping the future. As a contributor to The Brighter Side of News, he focuses on positive and transformative advancements in AI, technology, physics, engineering, robotics and space science. Joshua is currently working towards a Bachelor of Science in Business Administration at the University of California, Berkeley. He combines his academic background with a talent for storytelling, making complex scientific discoveries engaging and accessible. His work highlights the innovators behind the ideas, bringing readers closer to the people driving progress.