Major discovery about the origins of matter in the universe
The early universe was a scorching 250,000 times hotter than the core of our sun, far too intense to form the protons and neutrons.
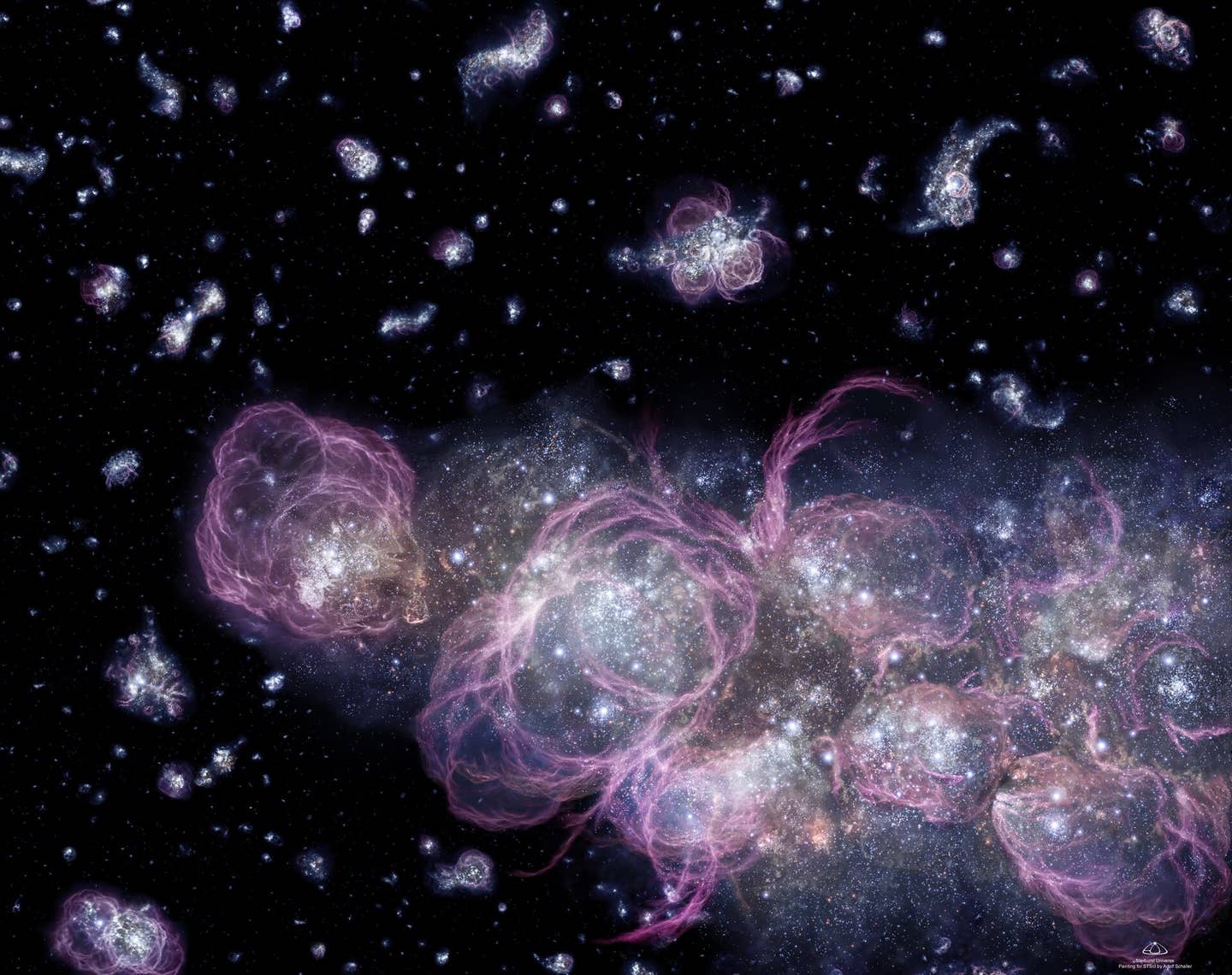
This discovery clarifies how much matter formed in the first few fractions of a second after the Big Bang versus how much emerged from later reactions as the universe expanded. (CREDIT: ESA)
The early universe was a scorching 250,000 times hotter than the core of our sun, far too intense to form the protons and neutrons that constitute everyday matter. To replicate these extreme conditions, scientists use particle accelerators, smashing atoms together at nearly the speed of light.
By measuring the resulting particle showers, you can gain insights into how matter formed. These particles emerge in various ways: some originate from the initial soup of quarks and gluons, while others result from subsequent reactions.
These later reactions began just 0.000001 seconds after the Big Bang, when quark-based composite particles started to interact with each other. A recent calculation has shown that up to 70% of some measured particles come from these subsequent reactions rather than from the early universe's initial reactions. This research, published in Physics Letters B, enhances our understanding of matter's origins.
This discovery clarifies how much matter formed in the first few fractions of a second after the Big Bang versus how much emerged from later reactions as the universe expanded. It suggests that a significant portion of the matter around us formed later than previously thought.
For accurate results in collider experiments, scientists must discount particles formed in these subsequent reactions, focusing only on those from the initial subatomic soup to reveal the universe's early conditions.
Related Stories
In the 1990s, physicists recognized that certain particles form in significant numbers from these later reactions. For example, D mesons can interact to create a rare particle called charmonium. However, there was no consensus on the importance of this effect because charmonium is rare and challenging to measure. Recent experiments have provided new data on how many charmonium and D mesons colliders produce.
Using this new data, physicists from Yale University and Duke University calculated the effect's strength, finding it much more significant than anticipated. Over 70% of the charmonium measured could be formed in these reactions.
As the hot soup of subatomic particles cools, it expands in a fireball. This process happens in less than one-hundredth of the time it takes for light to cross an atom, making it difficult to pinpoint how the fireball expands. However, the new calculation indicates that knowing the exact details of this expansion isn't crucial. The collisions produce a substantial amount of charmonium regardless.
This new finding brings science one step closer to understanding the origins of matter. It underscores the importance of differentiating between particles formed in the early universe and those from later reactions. This distinction is vital for accurately interpreting results from collider experiments and for enhancing our comprehension of the universe's formative moments.
The implications of this research are profound. By identifying how much matter around us formed later than expected, you gain a better grasp of the universe's evolution. This knowledge can refine the methods used in particle physics and improve the accuracy of experimental results.
The work of these physicists not only advances our understanding of the early universe but also shapes the future of research in this field.
Note: Materials provided above by The Brighter Side of News. Content may be edited for style and length.
Like these kind of feel good stories? Get The Brighter Side of News' newsletter.
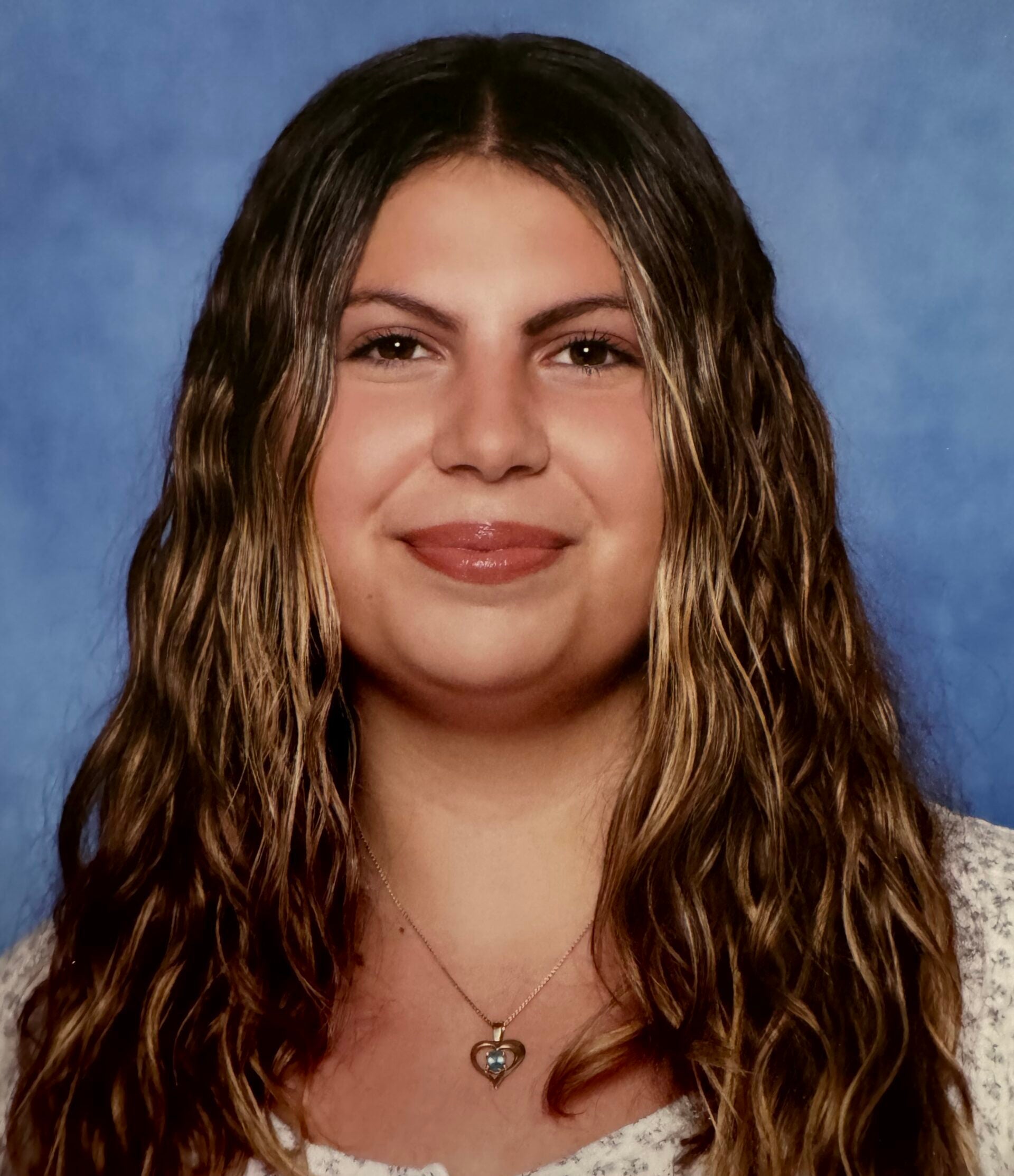
Rebecca Shavit
Science & Technology Journalist | Innovation Storyteller
Based in Los Angeles, Rebecca Shavit is a dedicated science and technology journalist who writes for The Brighter Side of News, an online publication committed to highlighting positive and transformative stories from around the world. With a passion for uncovering groundbreaking discoveries and innovations, she brings to light the scientific advancements shaping a better future. Her reporting spans a wide range of topics, from cutting-edge medical breakthroughs and artificial intelligence to green technology and space exploration. With a keen ability to translate complex concepts into engaging and accessible stories, she makes science and innovation relatable to a broad audience.