How the Earth and moon formed, explained
Scientists can use modern rocks, moon samples and meteorites to figure out when and how the Earth and moon formed over 4.6 billion years ago
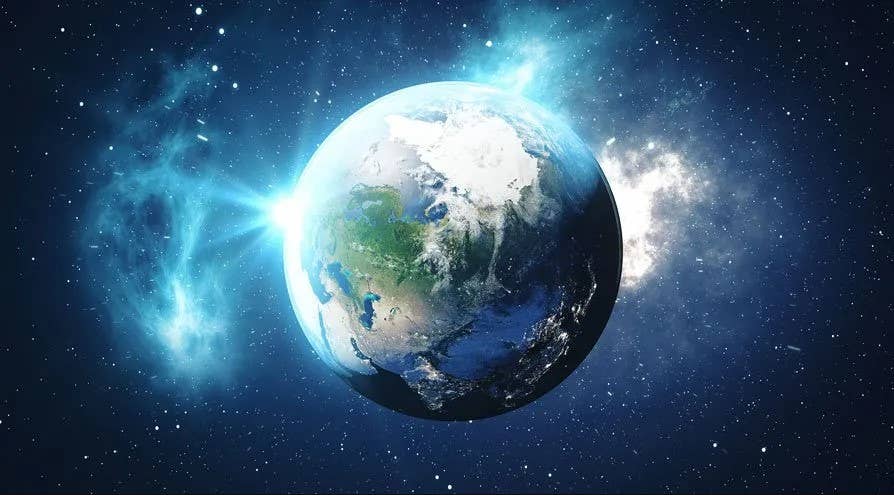
[July 3, 2022: Sasha Warren, University of Chicago]
Scientists can use modern rocks, moon samples and meteorites to figure out when and how the Earth and moon formed. (CREDIT: Wikimedia Commons)
The Earth formed over 4.6 billion years ago out of a mixture of dust and gas around the young sun. It grew larger thanks to countless collisions between dust particles, asteroids, and other growing planets, including one last giant impact that threw enough rock, gas, and dust into space to form the moon.
Although the rocks that record the earliest parts of Earth’s history have been destroyed or deformed by more than four billion years of geology, scientists can use modern rocks, moon samples and meteorites to figure out when and how the Earth and moon formed, and what they might once have looked like.
How did the Earth and moon form?
The Earth, like all the other planets in the solar system, started out its life as a disc of dust and gas orbiting the young Sun. The dust particles were brought together by the forces of drag to form clumps of rock that grew into “planetesimals” tens to hundreds of miles across, and then to Mars-sized “protoplanets” by colliding with each other.
Earth grew to its final size through one last major collision with another Mars-sized object. This last collision, also known as the “moon-forming impact”, was so large that—in addition to adding lots of material to the Earth—there was enough energy to vaporize some of the rock and metal from both the proto-Earth and the impacting object. This vapor formed a disc around the Earth that eventually cooled and clumped together to become the moon.
We know this thanks to rigorous studies of meteorites and rock samples, including at the University of Chicago, in the 20th and 21st centuries.
Related Stories
Understanding how the Earth and moon formed is important for piecing together the history of the solar system and answering questions like how long planets take to form, what planets are made of, and what makes a planet suitable for life. This also guides planetary scientists in their search for other habitable (or inhabited!) worlds in our solar system and beyond!
This artist's concept shows a very young star encircled by a disk of gas and dust, the raw materials from which rocky planets such as Earth are thought to form. (CREDIT: NASA/JPL-Caltech)
How and when did the early Earth form?
Scientists now think the Earth’s story began around 4.6 billion years ago in a disk-shaped cloud of dust and gas rotating around the early sun, made up of material left behind after the sun’s formation.
Within this disk, gas and dust particles of different sizes orbited the sun at slightly different speeds, allowing them to bump into each other and stick together. Eventually, they grew from tiny dust grains into boulders, then into larger “planetesimals” that ranged from miles to hundreds of miles in diameter.
Because these planetesimals were larger than the boulders, they had strong enough gravity to pull neighboring planetesimals out of orbits and absorb them through collisions, enabling some planetesimals to grow bigger and bigger until they reached thousands of miles in diameter—about the size of the moon and Mars.
How do we know?
The key is meteorites. Meteorites bring many different types of material from all over the solar system to Earth where scientists can study them. These materials include chondrules—tiny pieces of dust and rock that have survived from before the planets formed—and pieces of asteroids and planetesimals left behind by the planet-building process. Radioactive elements like uranium and hafnium are trapped inside the minerals that make up these objects when they form, which allows planetary scientists to tell how old they are.
Using these measurements, and simulations of the physics of dust and planetesimal collisions, planetary scientists and astronomers have established that the dust-to-protoplanet process takes tens of millions of years.
But the final stage of planet formation in our solar system may have taken much longer - up to a hundred million years or so. This was not only the last major addition of material to the Earth, but also the event that formed the moon—and it’s one of the most debated parts of the story.
How did the moon form?
Several different formation theories for the moon have been proposed by scientists, The story that is best supported by all the available data, however, is that the moon formed during a giant impact between the proto-Earth, and another protoplanet roughly the size of Mars, sometimes known as “Theia.”
In this theory, the moon formed from the impact debris—a mixture of molten rock and hot gas—flung out into space by the impact, potentially forming a disk of material known as a “lunar synestia.”
Alternate theories suggested by scientists include:
the moon broke away from the Earth (“Fission theory”)
the moon formed elsewhere in the solar system and was captured by Earth’s gravity (“Capture theory”)
the Earth and moon formed from the protoplanetary disk at the same time (“Co-formation”)
How do we know?
Samples of rock from the moon, brought to Earth by lunar meteorites and the Apollo moon landings, can be used to understand the history of the Moon and its relationship to the Earth through the chemistry of their minerals.
Planetary scientists like Prof. Nicolas Dauphas and Prof. Andy Davis in the Geophysical Sciences Department at the University of Chicago make precise measurements of lunar samples to determine exactly what they’re made of, and identify the chemical fingerprints of different geological processes like the melting and mixing of rocks and the evaporation of gasses.
The first big clue about where the moon came from comes from oxygen. Oxygen, like many other elements, is able to exist in multiple forms, known as isotopes. Different types of meteorites coming from the asteroids leftover in the Solar system after planet formation have different proportions of each of these oxygen isotopes. So, by measuring the oxygen isotopes of a given planet, planetary scientists can calculate the different types of asteroid that collided to form the planet. Lunar samples have a very similar oxygen isotope makeup to the Earth.
This artist's concept shows a celestial body about the size of our moon slamming at great speed into a body the size of Mercury. NASA's Spitzer Space Telescope found evidence that a high-speed collision of this sort occurred a few thousand years ago around a young star, called HD 172555, still in the early stages of planet formation. The star is about 100 light-years from Earth. (CREDIT: NASA/JPL-Caltech)
Some scientists believe that the oxygen isotopes are because the object that hit Earth was made up of the same mixture of meteorites as the Earth itself, potentially suggesting that the impactor planet formed close by in the solar system.
Other scientists propose that after the impact all of the oxygen was able to move around in the hot vapor surrounding the Earth and moon, mixing up all the different oxygen isotopes and erasing any original differences between the Earth and Theia.
However, there are many differences between the chemistry of the Earth and the moon, too. At the high temperatures reached during planetary impacts, many elements that we are not used to thinking of as gasses - like potassium, zinc, and sodium - can exist as vapor. The concentrations of these “volatile” elements are much lower in lunar rocks than in rocks on Earth.
One possibility is that the hot impact debris had a long time to evaporate these elements before it clumped together to form the moon. Another is that when the moon formed it started off very hot with a deep magma ocean - like the Earth - and the low gravity and lack of atmosphere on the moon allowed volatile elements that wouldn’t escape from a larger object to evaporate into space.
Both of these pieces of evidence are hard to explain without a giant impact. An impact origin for the moon provides the high temperatures needed to explain the lack of potassium, zinc, and sodium on the moon, and also an opportunity for lots of mixing between the proto-Earth and the material that would become the moon. But when did this impact take place?
When did the Moon form?
Scientists believe the moon formed during a giant impact about 60-175 million years after the solar system was born. To arrive at this estimate, they can use rocks from Earth.
As large planetesimals grow, heat released by repeated impacts and the radioactive decay of elements inside their minerals -- enough to cause melting. This allows materials with different densities to separate, with metals like iron and nickel sinking to the inside to form a core and lighter rocks “floating” on top.
A sample of lunar rock brought back by the Apollo 15 mission. Click here to expand image. (CREDIT: NASA)
By the time of the moon-forming impact, the Earth was already separated into these rock and metal layers. However, the intense force and heat of the impact re-melted the proto-Earth, re-mixing the separated rock and metal. After this mixing, the Earth was still hot enough for separation to occur again and form new rock and metal layers - this is the key to dating when the moon formed!
When rock and metal mix, they are able to swap certain elements. Elements like hafnium prefer to be mixed in with rock than with metal. Hafnium decays over about 10 million years to form tungsten. The first time the Earth cooled and separated into rock and metal layers was early in the solar system’s history, so lots of hafnium was present in the Earth’s rocky layer because it hadn’t had time to decay to tungsten yet. By the time the moon-forming impact occurred, much of this early hafnium had decayed to tungsten. Elements like tungsten prefer to be mixed in with metal, so when the impact remixed the Earth, the newly formed tungsten sank into the metal core. This created a rocky outer layer with a lower concentration of hafnium than before, and a metal core with much more tungsten in it.
Today, all of the hafnium is gone because it has a short half-life compared to the age of the Earth. However, not all is lost - this makes it very useful for working out the timing of events in the first hundred million years of the solar system’s history. The concentration of tungsten in Earth’s rocks depends on when the most recent separation into rock and metal layers occurred. The concentration of tungsten in Earth’s rocks is too low to be explained by the metal and rock separating early on, which means something must have re-mixed the Earth’s layers. The best explanation for the heat and energy needed to do this is a giant impact about 60-175 million years after the solar system was born.
What did the early Earth look like?
After the moon-forming impact, Earth was a very different planet from the world we see today! Where the present-day Earth has oceans covering much of its surface, the early Earth was covered in a magma ocean - a layer of molten rock hundreds of miles deep melted by the energy released during the collision. Any water present would only exist as water vapor in the atmosphere.
If that wasn’t enough, the early sun was also far more active than it is today, blasting the entire solar system with UV radiation energetic enough to evaporate entire atmospheres.
Over time, after the magma ocean cooled enough to form a solid surface, Earth’s atmosphere was replenished by volcanic eruptions, as well as water and other gasses delivered by comets and meteorites crashing into the surface.
This was also the first step towards our planet developing plate tectonics. Plate tectonics describes the giant “plates” of crust that slowly move around Earth’s surface over hundreds of millions of years; it not only produces new rocks at volcanoes where the plates are moving apart, but can also recycle rocks from the Earth’s surface and atmosphere back into the interior where plates are coming together. This process - known as “subduction” - carries rocks, water, and carbon dioxide trapped in minerals back into the Earth’s interior where they can drive future volcanic eruptions, continuing the plate tectonic cycle.
Some planetary scientists believe that plate tectonics is essential for a planet to develop life. This is because the repeated production and destruction of crust by plate tectonics both releases carbon dioxide to the atmosphere and removes it, helping keep temperatures on Earth similar (and comfortable for microbes, fish, and humans!) over billions of years.
Whether a planet has plate tectonics is much more complicated than just having a solid surface, though, and might also depend on the types and amount of different asteroids, planetesimals, and protoplanets that the Earth is made of because of the way different chemicals and minerals can change how planet interiors behave over billions of years.
What did the early moon look like?
Most of us picture the moon as a desolate, grey place with craters and not much else, but it was surprisingly geologically active for much of its history. Like the Earth, the moon started off with a thick layer of molten rock on its surface.
Unlike the Earth, though, the moon’s surface did not cool to form tectonic plates. Instead, it has a thick crust made up almost entirely of a light-colored mineral called feldspar. Feldspar is the main material that makes up the bright areas we can see on the moon today, also known as the lunar highlands. The feldspar crystalized as the magma ocean cooled, and was light enough to float to the moon’s surface, on top of other minerals and the remaining magma. (Planetary scientists can use the fact that this feldspar crust formed on the moon but not on the Earth to try and work out differences in the early chemistry and cooling conditions between the two objects to learn more about the moon’s formation.)
The formation of a feldspar crust didn’t mark the end of geological activity on the moon, though. The heat leftover by the impact, as well as more heat contributed by radioactive elements, was able to melt rock deep within the noon to fuel volcanoes on its surface. This melting produced basalt, a kind of dark-colored rock commonly found at volcanoes on Earth today in places like Hawaii and Iceland. The basalt spilled out over hundreds of kilometers across the moon’s surface, forming “mare” (meaning “seas” in Latin) up to a mile thick. These mare cover around 16% of the moon’s surface and are visible with the naked eye as the dark patches on the moon.
You can visually see the moon's geologic history written in dark basalt patches from ancient volcanoes in contrast to the lighter crust made out of feldspar. (CREDIT: NASA)
Planetary scientists can tell that the basalt mare are younger than the feldspar highlands using the number of craters on the different surfaces. The mare have fewer craters on their top surfaces than the highlands because they have had less time to be hit by asteroids and meteorites. The youngest mare are thought to be only 1.1 billion years old, which means volcanoes were still erupting on the moon two billion years after the earliest accepted evidence for life on Earth!
Another feature of the early moon was its orbit. Today, the moon is moving about 1.5 inches further away from the Earth every year. Planetary scientists have calculated the distance between the Earth and the Moon backwards in time and found that the moon used to be seventeen times closer (14,000 miles vs. 250,000 miles) when it formed.
This changing distance between the Earth and the moon is an important clue about the details of the moon-forming impact because changing the size, speed and angles of approach of impactors in moon formation simulations changes the orbit of the final Earth-moon system. Planetary scientists need to find an impact simulation that can not only match the moon’s chemistry, but also how far it was from the Earth and how fast it was initially spinning.
What questions remain?
Although scientists agree that the moon formed because of an impact, the details of the impact are still up for debate. Scientists still don’t agree on how big the impacting object was, how fast it was travelling, what it was made from, or even whether we should be calling it “Theia”. Some scientists even argue that multiple impacts might have formed the Moon, instead of just one!
Lab experiments help scientists better understand what happens to different rock types and elements under the extreme conditions of large impact events. Prof. Nicolas Dauphas’ research group at UChicago evaporates metals under a vacuum to simulate the conditions present in the cloud of impact debris to try and explain why lunar rocks have so much less of elements like sodium, zinc, and potassium compared to the Earth.
Some experiments can be too large for the lab, though, so computer simulations are also used to investigate the moon-forming impact. These simulations enable scientists to virtually smash together the proto-Earth and different types of planetesimals at many speeds and angles to figure out what combinations of properties are able to form a moon with the size and orbit we see today.
In the future, new samples from the moon could give planetary scientists a wider range of different lunar rock types to work with. This is important because new samples might record different pieces of the moon’s history that aren’t present in the existing lunar rocks brought back by the Apollo missions. As measurement techniques improve, planetary scientists will also be able to measure new chemical signatures in lunar rocks, and improve upon existing measurements. The more measurements scientists have, the more ways they can test different theories about how our moon formed, its relationship to the Earth, and perhaps even how moons might be born around other planets far beyond our solar system!
Note: Materials provided above by University of Chicago. Content may be edited for style and length.
Like these kind of feel good stories? Get the Brighter Side of News' newsletter.
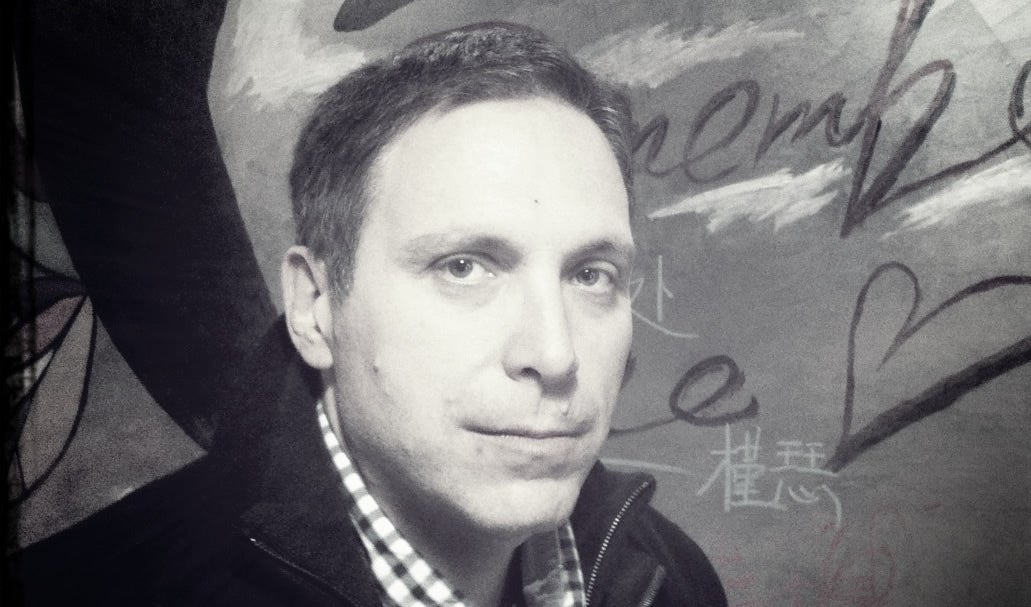