How does the Earth generate its magnetic field?
Iron, the most abundant element by mass on Earth, reveals a fascinating complexity under extreme conditions of temperature and pressure.
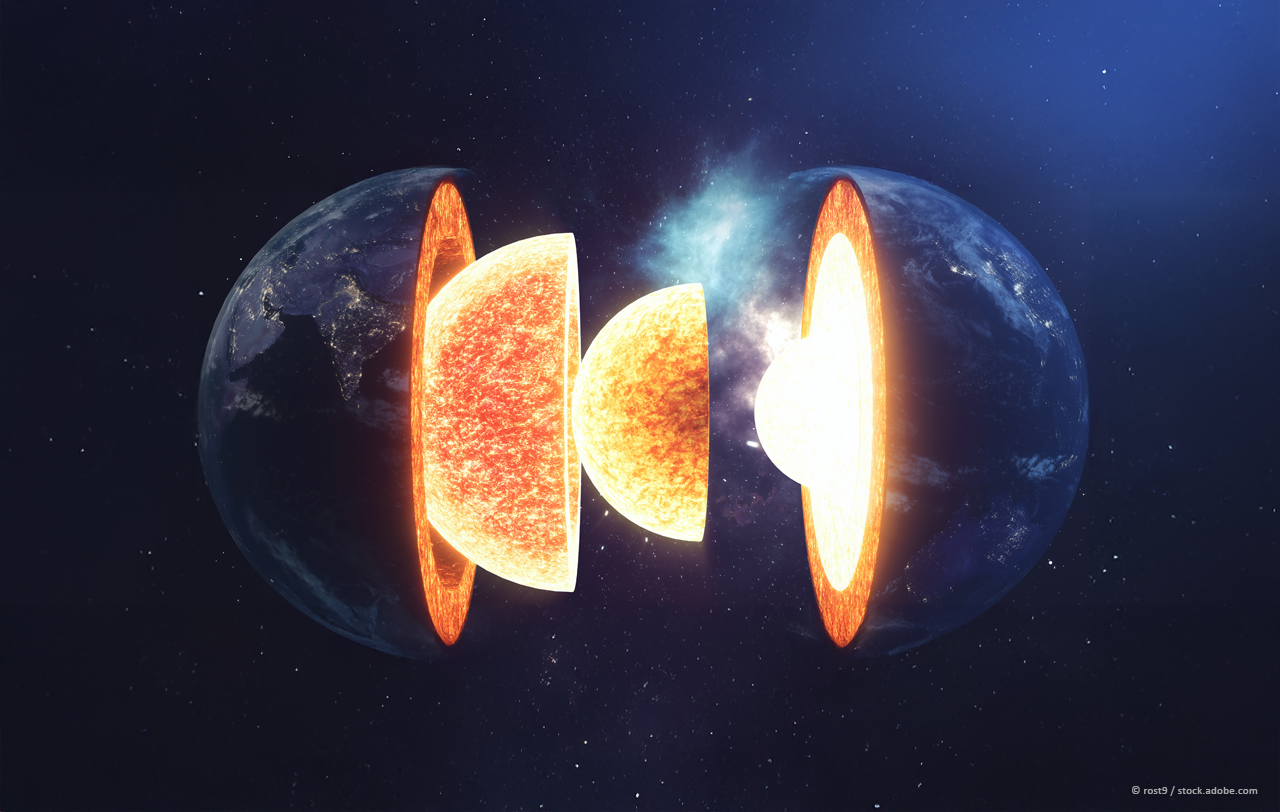
The Earth has a shell-like structure. It is divided into a core (inner and outer core), a mantle (lower and upper mantle) and the earth’s crust. The outer core and the lower mantle are highlighted in the center of the illustration. (CREDIT: rost9/stock.adobe.com)
Iron, the most abundant element by mass on Earth, reveals a fascinating complexity under extreme conditions of temperature and pressure. For thousands of years, humans have relied on iron for tools, construction, and even advanced medical technologies like drug delivery systems.
Its significance, however, extends far beyond its terrestrial applications. At the heart of our planet, iron plays a crucial role in geophysical and geochemical processes that sustain life on Earth.
Deep within the Earth, at pressures nearing 350 gigapascals and temperatures exceeding 6000 Kelvin, iron flows in the planet’s core. This dynamic movement generates Earth's magnetic field, a protective shield against harmful solar radiation and cosmic particles.
This magnetic field also influences plate tectonics and mantle convection, underlining the central role iron plays in Earth's habitability. Despite its importance, the exact mechanisms driving Earth's magnetic field remain elusive.
Scientists generally attribute this phenomenon to the geodynamo effect. Here, the convective movement of electrically charged, molten iron in the outer core produces electrical currents, generating the magnetic field.
Understanding this geodynamo mechanism requires detailed knowledge of iron’s properties under extreme conditions, including its phase transitions, melting points, and transport properties such as thermal and electrical conductivity.
Experimental studies have advanced the understanding of iron’s behavior. Early experiments employed shock and compressive waves to explore iron’s phases at low pressure, gradually extending to conditions similar to those in Earth's core.
Recent advances, such as laser-driven shocks and dynamic compression techniques, have expanded this knowledge further. However, challenges persist in measuring critical properties like electrical and thermal conductivity under these extreme conditions. Discrepancies in experimental results have fueled debates, underscoring the need for computational methods to fill the gaps.
Related Stories
Computational simulations have become indispensable in this endeavor. Classical molecular dynamics (MD) simulations, enhanced by machine learning techniques, represent the state of the art in modeling iron’s behavior at the atomic level.
These methods have yielded highly accurate interatomic potentials based on first-principles calculations. However, earlier models often overlooked magnetic effects, leading to inaccurate predictions for properties such as heat capacity.
To address this, researchers have integrated magnetic properties into MD simulations. Recent developments include molecular-spin dynamics (MSD) simulations that incorporate both atomic motion and magnetic behavior.
These advanced models achieve quantum-level accuracy by partitioning magnetic and non-magnetic contributions to the potential energy surface. By simulating iron’s behavior at Earth-core conditions, researchers have mapped its polymorphic phases and melting line with unprecedented precision.
One significant breakthrough comes from a team at the Helmholtz-Zentrum Dresden-Rossendorf, Sandia National Laboratories, and the French Alternative Energies and Atomic Energy Commission.
Their work integrates spin dynamics with molecular dynamics to analyze interactions between mechanical and magnetic properties of iron under high-pressure and high-temperature conditions. Using machine learning, the team developed precise force fields to model these interactions, enabling simulations on unprecedented spatial and temporal scales.
These simulations revealed the stabilizing effects of magnetic properties on iron’s phases under extreme conditions. For instance, the body-centered cubic (bcc) phase of iron, previously hypothesized but not experimentally observed under core-like conditions, emerged in the simulations. If confirmed, this phase could provide critical insights into the geodynamo effect and Earth’s magnetic field.
The implications of this research extend beyond geophysics. The simulation techniques hold promise for developing innovative technologies.
For example, spin-based simulations could accelerate the design of neuromorphic computing devices, which mimic brain-like processing for energy-efficient artificial intelligence. These methods could also revolutionize data storage technologies by modeling magnetic domains in nanowires, potentially surpassing the speed and efficiency of current systems.
Despite these advancements, questions about Earth’s core persist. Seismic wave studies suggest the core contains elements other than iron, which may influence its behavior. Addressing these uncertainties will require further refinement of experimental and computational methods.
Combining these approaches could unlock new knowledge about Earth’s interior and its magnetic field, while paving the way for technological breakthroughs inspired by the unique properties of iron.
Note: Materials provided above by The Brighter Side of News. Content may be edited for style and length.
Like these kind of feel good stories? Get The Brighter Side of News' newsletter.
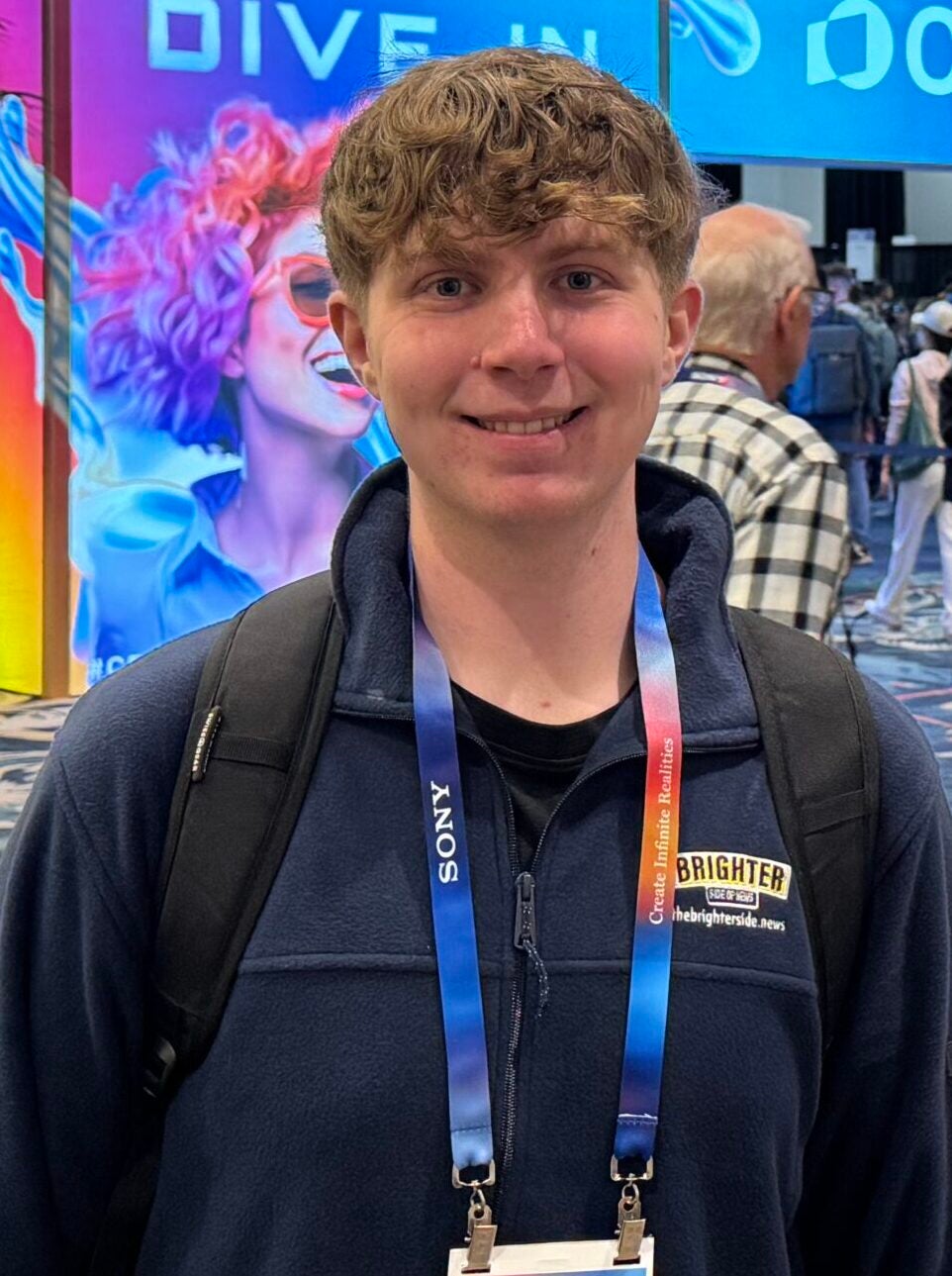
Joshua Shavit
Science & Technology Writer | AI and Robotics Reporter
Joshua Shavit is a Los Angeles-based science and technology writer with a passion for exploring the breakthroughs shaping the future. As a contributor to The Brighter Side of News, he focuses on positive and transformative advancements in AI, technology, physics, engineering, robotics and space science. Joshua is currently working towards a Bachelor of Science in Business Administration at the University of California, Berkeley. He combines his academic background with a talent for storytelling, making complex scientific discoveries engaging and accessible. His work highlights the innovators behind the ideas, bringing readers closer to the people driving progress.