Historic breakthrough in quantum mechanics and gravity research
General relativity and quantum mechanics are two of the most important scientific breakthroughs of the 20th century. However, we still do not know how gravity might be quantized.
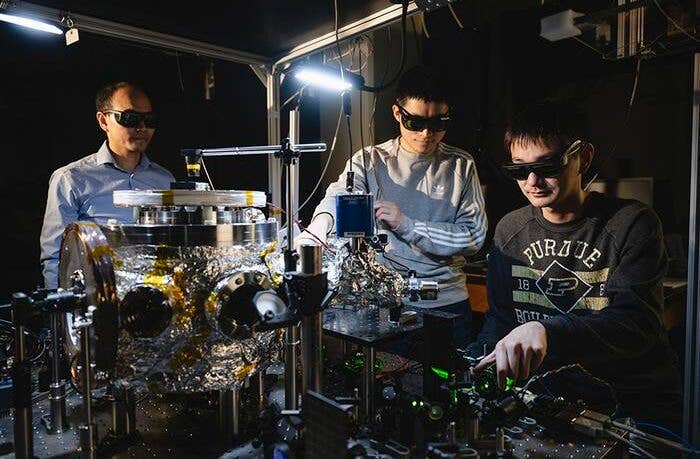
Prof. Tongcang Li (left), Dr. Yuanbin Jin (middle) and Kunhong Shen perform experiments with levitated and rotating fluorescent diamonds at Purdue University. (CREDIT: Purdue University / Charles Jischke)
Physicists at Purdue University have achieved a significant breakthrough by creating a unique experimental setup involving levitated fluorescent nanodiamonds. These tiny diamonds are not just floating in a vacuum; they’re spinning at incredibly high speeds, emitting and scattering multicolored lights as they rotate.
This research, led by Tongcang Li, a professor at Purdue, marks an exciting advancement in the study of rotating quantum systems and levitodynamics, with their results published in Nature Communications. Reviewers have hailed the work as “arguably a groundbreaking moment” and “a new milestone for the levitated optomechanics community.”
Li, who is also a member of the Purdue Quantum Science and Engineering Institute, explains the significance of their work. "Inside these floating diamonds, there are spin qubits that allow us to make precise measurements and explore the mysterious relationship between quantum mechanics and gravity.
Previous experiments faced challenges in preventing the diamonds' loss in vacuum and in reading out the spin qubits. However, in our work, we successfully levitated a diamond in a high vacuum using a special ion trap and, for the first time, observed and controlled the behavior of the spin qubits inside the levitated diamond."
By rotating these diamonds at speeds of up to 1.2 billion times per minute, the team could observe the unique effect of rotation on the spin qubits, known as the Berry phase. Li highlights that this achievement deepens our understanding of the quantum world.
The nanodiamonds used in the experiment have an average diameter of about 750 nanometers. These diamonds were produced through a high-pressure, high-temperature synthesis process, followed by irradiation with high-energy electrons to create nitrogen-vacancy color centers, which host the electron spin qubits.
When illuminated by a green laser, the diamonds emit red light, which is used to read out their electron spin states. Additionally, an infrared laser is used to monitor the diamonds' rotation. As the nanodiamonds rotate, they scatter infrared light in different directions, similar to how a disco ball scatters light, which provides information about the rotation.
Related Stories
The research team primarily consists of members from Li’s research group at Purdue, including postdoc Yuanbin Jin, PhD students Kunhong Shen and Xingyu Gao, and recent PhD graduate Peng Ju. Jin, Shen, and Ju were instrumental in conceiving and designing the project, while Jin and Shen built the experimental setup.
The team collectively discussed the results, with contributions from Alejandro Grine of Sandia National Laboratories and Chong Zu from Washington University in St. Louis, who offered suggestions to improve the experiment and manuscript.
Jin explains the technical aspects of their work: “For the design of our integrated surface ion trap, we used commercial software to perform 3D simulations. We optimized the design by calculating the trapping position and microwave transmittance using different parameters. We also added extra electrodes to conveniently control the motion of a levitated diamond. The surface ion trap is fabricated on a sapphire wafer using photolithography, and a 300-nanometer-thick gold layer is deposited on the wafer to create the electrodes.”
Shen adds that they have the capability to adjust the spinning direction of the diamonds. "We can change the spinning direction by adjusting the driving voltage. The levitated diamond can rotate around the z-axis, either clockwise or counterclockwise, depending on our driving signal. Without the driving signal, the diamond spins in multiple directions, like a ball of yarn.”
Levitated nanodiamonds with embedded spin qubits are promising for precision measurements and could help create large quantum superpositions to test the limits of quantum mechanics and gravity.
Li underscores the importance of this work: “General relativity and quantum mechanics are two of the most important scientific breakthroughs of the 20th century. However, we still do not know how gravity might be quantized. Studying quantum gravity experimentally would be a tremendous breakthrough. Rotating diamonds with embedded spin qubits provide a platform to explore the coupling between mechanical motion and quantum spins.”
This discovery could have broader implications for industrial applications. Li mentions that levitated micro- and nano-scale particles in vacuum could serve as excellent accelerometers and electric field sensors. For instance, the US Air Force Research Laboratory is already using optically-levitated nanoparticles to address critical issues in navigation and communication.
Li also notes that Purdue University is well-equipped for such research. "At Purdue, we have state-of-the-art facilities for our work in levitated optomechanics. We have two specialized, home-built systems dedicated to this area of study. Additionally, we have access to the Birck Nanotechnology Center, which enables us to fabricate and characterize the integrated surface ion trap on campus."
"We’re fortunate to have talented students and postdocs capable of conducting cutting-edge research. My group has been working in this field for ten years, and our extensive experience has allowed us to make rapid progress," he continued.
This research is a part of Purdue's broader focus on quantum technologies, a key pillar of the Purdue Computes initiative, which emphasizes the university’s commitment to advancing technological and computational research.
Note: Materials provided above by The Brighter Side of News. Content may be edited for style and length.
Like these kind of feel good stories? Get The Brighter Side of News' newsletter.
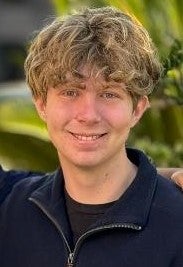