Groundbreaking research redefines what it means to be alive or dead
New research redefines cellular death with a mathematical model, offering insights into life, death, and their implications for biology and medicine.
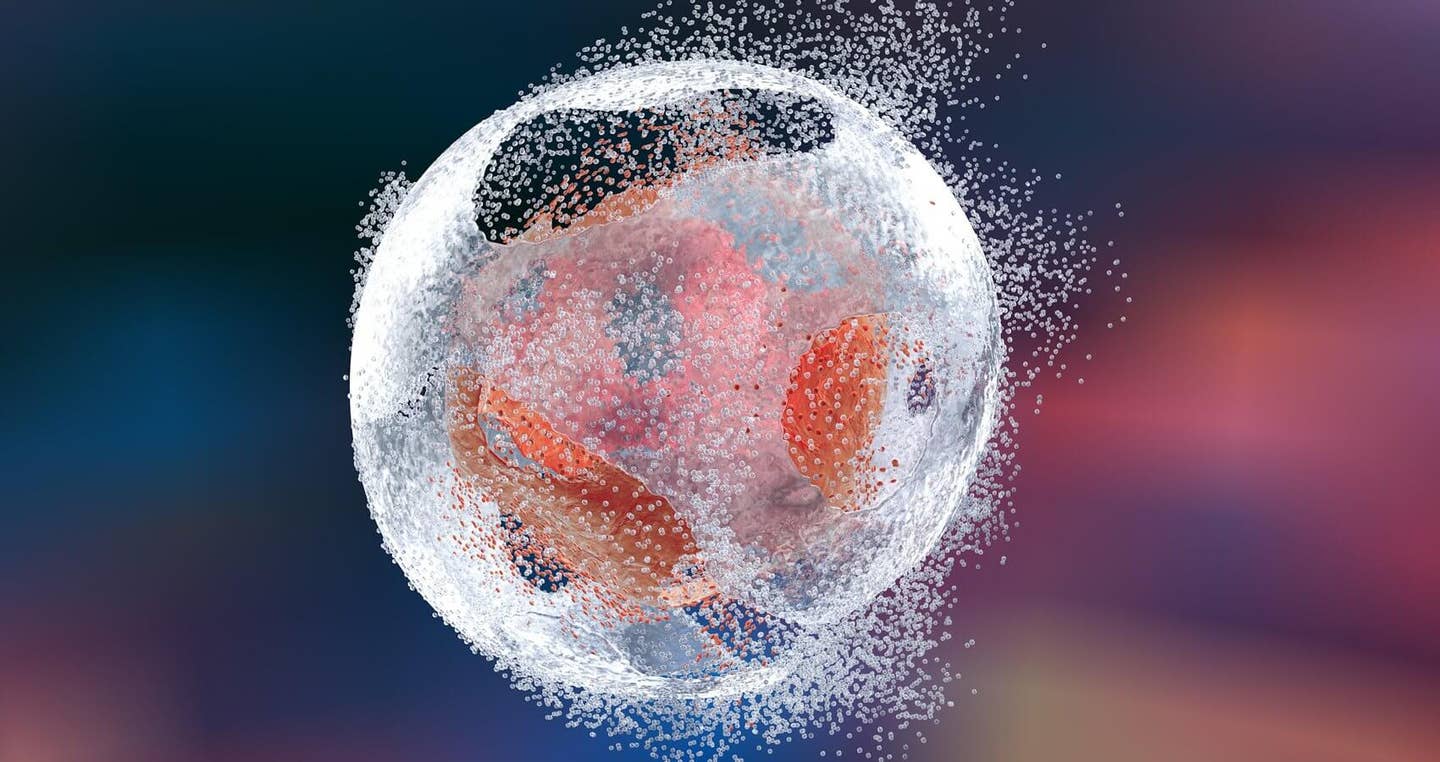
Scientists unveil a mathematical model redefining cellular death, bridging experimental data and theory, and unlocking new possibilities for biology and medicine. (CREDIT: CC BY-SA 4.0)
Death, a universal and inevitable phenomenon, serves as a cornerstone in the study of biological systems. Yet, the precise definition of cellular death, particularly at the microscopic level, remains a topic of contention among scientists.
While the boundaries between life and death seem intuitive on the surface, they blur significantly when examined in the context of microorganisms like Escherichia coli and yeast.
The absence of a universally accepted definition of cell death has long impeded progress in microbial studies. This ambiguity becomes particularly problematic when researchers encounter states such as "viable but non-culturable" (VBNC), where cells exist in a dormant condition, defying classification as either alive or dead.
Such definitional challenges have parallels in other fields, like bacterial persistence, where consensus definitions were necessary to prevent research stagnation. Without clarity, fundamental questions—What is death? What criteria define a dead cell?—remain unresolved, hindering advancements in cell death research.
Unlike other biological phenomena, microbial death research has lagged behind in integrating experimental data with theoretical modeling.
Theoretical approaches in this field are largely confined to estimating death rates and their effects on population dynamics. A robust mathematical framework to delineate death and evaluate cellular viability has become an urgent scientific necessity.
A New Approach to Death
Researchers at the University of Tokyo propose a groundbreaking mathematical definition of cellular death, aiming to bridge the gap between experimental data and theoretical understanding.
Related Stories
Assistant Professor Yusuke Himeoka and his team have introduced a model that defines death in terms of controllability—whether a potentially dead cell can return to a predefined "representative state of living."
This concept shifts focus from observable characteristics, such as metabolic activity, to a system's ability to regain functionality. Their method relies on enzymatic reactions, leveraging a key property: enzymes accelerate reaction rates without altering equilibrium states.
By focusing on these reactions, the team developed "stoichiometric rays," a computational tool that quantifies the life-death boundary based on biochemical pathways and thermodynamics.
Himeoka’s approach draws inspiration from the second law of thermodynamics, which dictates that systems naturally progress from order to disorder. This principle underpins the irreversible nature of many biochemical processes. Dead states, as defined by this model, are those from which cells cannot return to a living state, regardless of biochemical intervention.
The stoichiometric rays method enables researchers to evaluate global controllability in enzymatic systems efficiently. By simulating various cellular states, scientists can identify whether a cell's metabolic network can be redirected toward a living state. This quantitative approach provides unprecedented insight into the dynamics of life and death at the cellular level.
Implications for Biology and Medicine
This new framework has far-reaching implications. By establishing a mathematical definition of death, researchers can better understand how cellular systems fail. It opens avenues for exploring ways to reverse cellular death under controlled conditions, which could revolutionize fields like regenerative medicine and tissue engineering.
“My long-term scientific goal is to understand the inherent difference between life and nonlife, mathematically,” said Himeoka. “Why is the transition from nonlife to life so difficult, while the other way around is so easy? Our aim was to develop a computational method to quantify this life-death boundary.”
The study also sheds light on the concept of autonomy in living systems. Autonomic processes—where cellular machinery such as proteins self-regulate—pose challenges for the current model. Himeoka envisions extending their method to address these complexities.
“We naively believe that death is irreversible, but it’s not so trivial and does not have to be the case,” he explained. “Should death come more under our control, our understanding of life and society will change completely. To understand death is crucial, not just in science but also in terms of social implications.”
Computational Biology: A Promising Future
Advancements in computational biology have paved the way for large-scale simulations of cellular processes. Technologies such as machine learning and whole-cell kinetic modeling offer new tools to study life and death.
These innovations enable scientists to model scenarios where cells undergo both growth and death, exploring the biochemical pathways that determine these states.
By integrating computational methods with experimental data, researchers can uncover the design principles governing cellular systems. This fusion of theory and practice promises to propel cell death research into a new era, with potential applications ranging from disease treatment to synthetic biology.
The integration of mathematical frameworks into biological research represents a significant step forward. By providing a precise definition of cellular death, Himeoka’s team addresses a fundamental question that has long eluded scientists.
Their work not only advances our understanding of mortality but also lays the foundation for transformative applications in medicine and biology.
In the long run, this research challenges conventional views on the irreversibility of death. It invites a deeper exploration of the life-death continuum, reshaping how we perceive the very essence of existence.
Note: Materials provided above by The Brighter Side of News. Content may be edited for style and length.
Like these kind of feel good stories? Get The Brighter Side of News' newsletter.
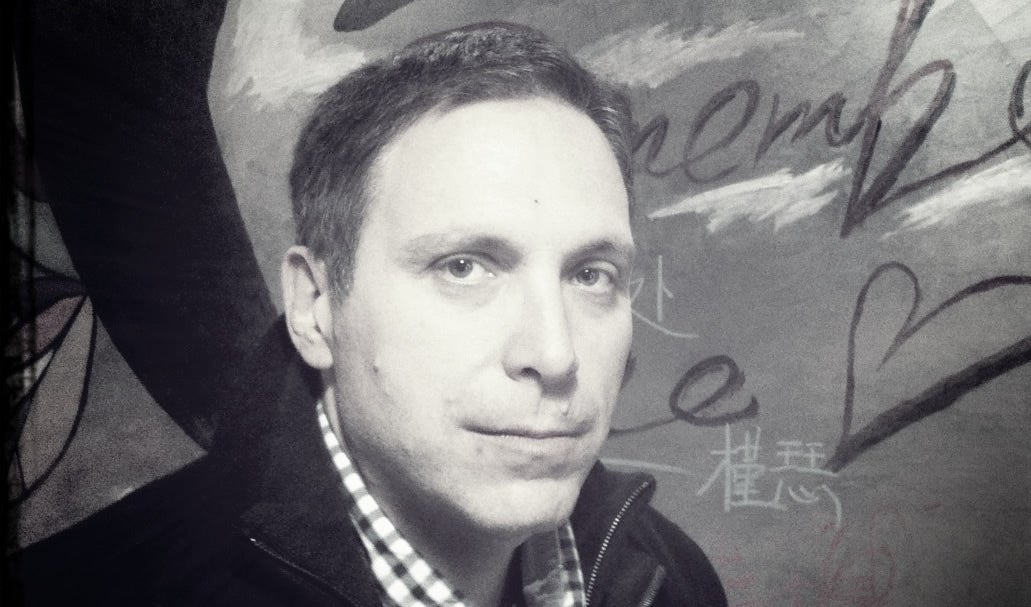