Groundbreaking new jet engine generates thrust directly from electricity
The combustion of these fuels powers vehicles, planes, and industrial equipment while contributing nearly 29% of greenhouse gas emissions
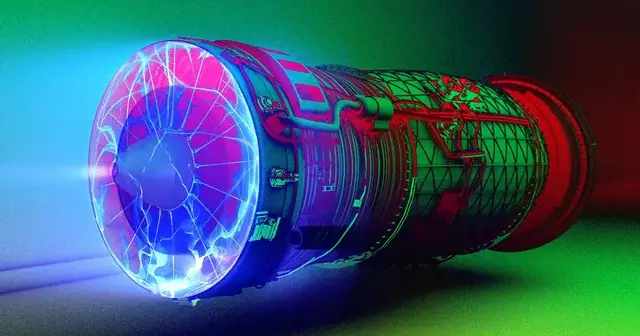
Researchers have developed a prototype jet engine powered by microwave air plasmas, offering thrust without fossil fuels. (CREDIT: CC BY-SA 4.0)
The global reliance on fossil fuels, particularly in transportation, has severe consequences for the environment and public health. Fossil fuels are a leading source of greenhouse gas emissions, driving climate change and impacting respiratory health.
A groundbreaking development from Wuhan University, however, hints at a future where jet propulsion depends entirely on electricity and air.
The Fossil Fuel Challenge
Transportation is vital for modern life, but its dependence on fossil fuels comes at a significant environmental cost. The combustion of these fuels powers vehicles, planes, and industrial equipment while contributing nearly 29% of greenhouse gas emissions, according to the Environmental Protection Agency. The urgent need for sustainable alternatives has spurred innovation.
Professor Jau Tang and his team at Wuhan University have developed a prototype jet engine powered by microwave air plasmas, offering thrust without fossil fuels. This revolutionary approach could transform air travel by eliminating carbon emissions.
“Our work aims to solve global warming problems by replacing fossil fuel combustion engines,” Tang explained. “With our design, there is no carbon emission to cause greenhouse effects and global warming.”
How Plasma Powers the Engine
Plasma, the fourth state of matter, consists of charged particles like ions and electrons. Found naturally in the sun and lightning, it can also be generated in controlled environments. Tang's engine harnesses this state of matter through a sophisticated process involving air compression and microwave technology.
Related Stories
Here’s how it works:
- Air Compression: The system starts by drawing in atmospheric air, which is then compressed to high pressures using a turbine compressor. This compressed air provides the necessary density for effective plasma generation.
- Microwave Ionization Chamber: The compressed air flows into a quartz tube fitted with a microwave ionization chamber. Microwaves, operating at 2.45 GHz—the same frequency used in microwave ovens—are directed into this chamber.
- Ionization: Inside the chamber, the high-frequency microwaves excite the air molecules, stripping electrons from the atoms and creating a plasma state. The resulting plasma reaches temperatures exceeding several thousand degrees Celsius.
- Jet Thrust Generation: The high-temperature plasma rapidly expands as it exits the ionization chamber. This expansion produces a jet thrust capable of lifting a 1-kilogram steel ball, demonstrating thrust comparable to conventional jet engines.
Tang’s approach differs from other plasma propulsion systems, such as those used by NASA. For example, NASA’s xenon-based plasma thrusters, like those on the Dawn spacecraft, work well in the vacuum of space but are ineffective in Earth’s atmosphere due to their low thrust output. Tang’s design overcomes this limitation by using atmospheric air, making it feasible for terrestrial and airborne applications.
The Road to Scalable Plasma Jets
While the prototype is promising, scaling it to power large aircraft presents unique challenges. The current design requires megawatt-level microwave sources and advanced energy storage systems capable of delivering continuous high power.
“For a large jumbo jet, development could take another decade,” Tang estimated. Scaling up involves integrating multiple plasma jet modules in a parallel configuration. This would increase the overall thrust while maintaining efficiency.
The prototype already achieves a jet pressure of 24,000 newtons per square meter with 400 watts of power, comparable to commercial aircraft engines. However, larger aircraft will require significantly higher power outputs, which demands advancements in battery technology.
Tang believes smaller-scale applications, such as heavy-duty drones or pilotless cargo planes, could become operational within five years. These aircraft would be ideal for logistics and delivery services, reducing emissions in the transportation sector.
However, even for these smaller applications, challenges remain. The high energy density required for sustained flight means that current battery technologies must evolve to be lighter and more efficient. Weight is a critical issue, as heavy batteries could negate the benefits of this zero-emission propulsion system.
Addressing Engineering Challenges
Another hurdle is thermal management. Plasma engines generate extreme heat, which can damage engine components over time. Tang’s team is investigating advanced materials and cooling systems to mitigate these effects.
“We still need to improve the engine’s efficiency and address the impact of high temperatures on the equipment,” Tang noted. “Managing the heat and ensuring durability under continuous operation are our next big challenges.”
Additionally, achieving stable and controlled thrust across different flight conditions is crucial. The team is optimizing the flow dynamics within the ionization chamber to ensure consistent performance.
A Vision for the Future of Air Travel
Despite the challenges, Tang remains optimistic. His research has garnered attention from the global scientific community, with many experts recognizing its potential to revolutionize aviation. If successful, plasma jet engines could lead to a new era of sustainable air travel, free from the environmental and geopolitical constraints of fossil fuels.
“Our results demonstrate that a microwave air plasma jet engine could be a viable alternative to conventional fossil fuel engines,” Tang said.
While it may take years before you see plasma-powered planes in the sky, the foundation is being laid for a future where aviation is cleaner, quieter, and more sustainable. Tang’s groundbreaking work not only promises a solution to climate change but also redefines what’s possible in propulsion technology.
Note: Materials provided above by The Brighter Side of News. Content may be edited for style and length.
Like these kind of feel good stories? Get The Brighter Side of News' newsletter.
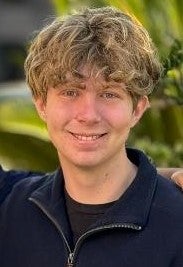