Generating “Blue Energy” where seawater and river water meet
Scientists have known since the 1950s that it is theoretically possible to generate electricity through the movement of water.
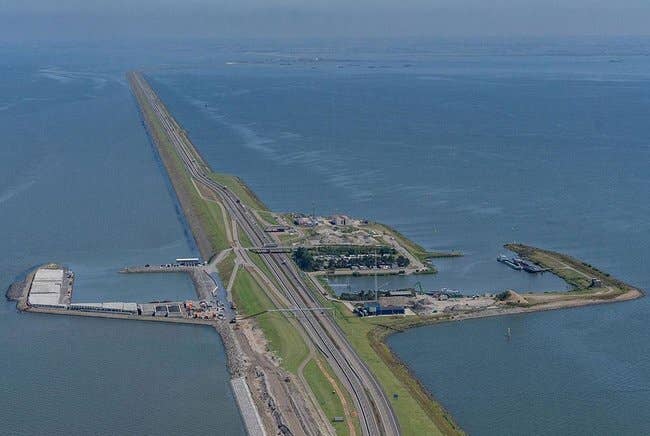
[Aug 15, 2022: Yao Meng, Tsinghua University Press]
(Credit: Tsinghua University Press)
Scientists have known since the 1950s that it is theoretically possible to generate electricity through the movement of water at the place where seawater and river water meet. This type of technology is called osmotic power generation or blue energy. Though prototypes of this technology have been built, research is still underway to prove that this technology is scalable and reliable.
In a literature review published in Nano Research Energy, researchers looked at the different types of materials that can be used in osmotic power generation.
“There are several locations on the earth where the seawater and river water are naturally mixed. The seawater contains positively charged ions such as sodium ions and negatively charged ions such as chloride ions. Utilizing electrostatically charged membranes that are selective to one ion species while blocking the other can generate electricity that can power electronic devices or be stored in batteries,” said paper author Javad Safaei, a researcher at the Centre for Clean Energy Technology at the University of Technology Sydney in Sydney, Australia.
Osmotic power generation is promising as an alternative to fossil fuels for several reasons. Salt water is readily available, the primary waste product of this technique is brackish water and, unlike wind and solar power, electricity produced through this method is not intermittent. Research suggests that around one terawatt, or one trillion watts, could be produced by this method every year. This is in comparison to the global solar output (710 gigawatts) and wind energy output (730 gigawatts), as of 2020.
Related Stories:
Because research is moving at such a fast pace, this literature review compared the different types of 2D materials that have been studied for use in osmotic power generation.
In order to generate electricity, the water will need to pass through a membrane. If there are two chambers of water with different salinity, the salt water will move through the membrane into the chamber with less salt water. Different types of materials with different properties and benefits can be used as this membrane.
The new review paper looked at how the materials were made, their physical characteristics, ion transportation properties, and their ability to generate power through osmosis.
A thin membrane is placed across two reservoirs with a salinity gradient that is connected via Ag/AgCl electrodes (a). The schematic diagram of a nanofluidic channel which is comprised of thin atomic layers with nanopores, placed on a supporting window (b). Typical I-V curves under an osmotic gradient (blue curve) and no osmotic gradient (green curve) (c). A thin 2D membrane with a nanopore that shows selective ion transportation (d). Reproduced with permission from Ref. [31], © Springer Nature Limited 2019. The diffusio-osmotic mechanism in a charged nanofluidic channel (e). Reproduced with permission from Ref. [40]. © Macmillan Publishers Limited 2017.
“Currently, these membranes are made by using a variety of materials. Amongst all, 2D materials stand out as the most promising candidate owing to their high ionic conductivities, high mechanical strength, large-scale production capabilities, and the ability to be formed into ultra-thin layers. Furthermore, several nanostructures with enhanced performances can be fabricated by using the hybrid of 2D materials with other nanomaterials,” said Safaei.
Through this review, researchers hope to raise awareness about osmotic power generation through 2D materials. “The most important message we have is to first introduce the concept of converting salt concentration difference of seawater/river water into electricity to a broader audience. We also hope to accelerate the research progress in this field by further specifying the focus on 2D materials, which are the most promising for making high-performance ion-conducting membranes,” said Safaei.
Researchers looked at the different types of materials that can be used in osmotic power generation. (Credit: Nano Research Energy, Tsinghua University Press)
Looking ahead, researchers are hoping to expand the scalability of osmotic power generation using 2D materials, but more research needs to be done.
“The 2D materials are currently the most promising classes of materials that can make practical devices that convert the chemical potentials of seawater/river water into electricity. We envision that further research into 2D materials makes practical salinity gradient power generation one step closer to reality,” said Safaei.
Cation-permselectivity of a 0.65 nm-thick MoS2 nanopore membrane (a). Top panel, simulation box for MD simulations showing MoS2 nanopore and the salts. Bottom panel, MD simulation results for potassium and chloride ions of different concentration gradients as a function of radial distance from the pore center (b). TEM-drilled MoS2 nanopore with a diameter of 5 nm (c). I-V curves of MoS2 nanopores with different pore sizes (black, 2 nm; red, 6 nm; blue, 25 nm) (d). The osmotic current and osmotic potential as a function of pore sizes (e). Reproduced with permission from Ref. [47], © Macmillan Publishers Limited, part of Springer Nature 2016. Schematic demonstration of the fabrication process for a nanoporous 2D carbon membrane using bottom-up synthesis route (f). Chemical structure of HPAHBC monomer (g). Atomic force microscopy (AFM) image of the membrane (inset, height profile of the membrane). Scale bar, 1 μm (h). Scanning electron microscopy (SEM) image of the membrane on a perforated substrate with an array of 2 µm diameter holes. Scale bar, 4 μm (i). HAADF-STEM image of the membrane showing the nanopores as dark regions on a bright background. (inset, pore size distribution) (j). Current density and power density profiles in 0.5 M/0.01 M NaCl (k). Reproduced with permission from Ref. [51], © Liu, X. et al. 2020. Schematic of ion transport through COF monolayer membrane (l). Simulated structure of ZnTPP-COF using DFT calculations (m). AFM image and height profile of ZnTPP-COF monolayer membrane (n). Large-scale (o) and high-resolution scanning tunnelling microscopy (STM) image of ZnTPP-COF membrane (p). Inset: corresponding fast Fourier transform images. The white dotted lines in (o) denote the grain boundaries and the white dotted rectangles in (p) represent nanopores. Current and power density profiles vs. external load resistance measured using artificial sea water and river water (q). Reproduced with permission from Ref. [52], © Yang, J. L. et al. 2022.
Guoxiu Wang at the Centre for Clean Energy at the University of Technology Sydney also contributed to this research.
For more green news stories check out our Green Impact section at The Brighter Side of News.
Note: Materials provided above by Tsinghua University Pres. Content may be edited for style and length.
Like these kind of feel good stories? Get the Brighter Side of News' newsletter.
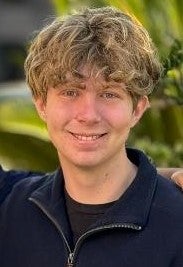