From gas giants to rocky worlds: Why planets in our solar system differ
Our solar system’s planets formed from the same nebula. Scientists explain how temperature, migration, and chemistry shaped their diversity.
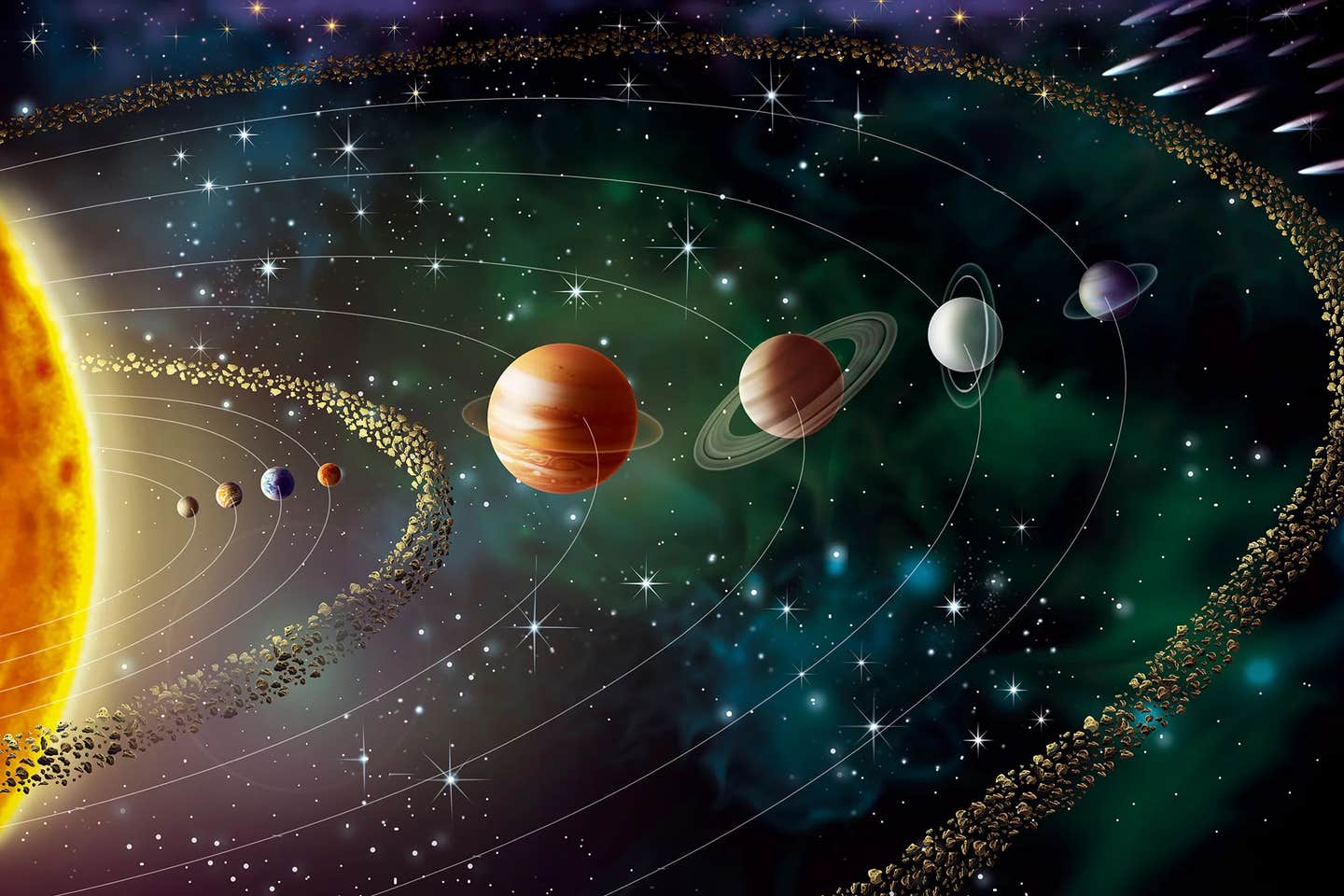
The formation of our solar system from a singular nebula raises an intriguing question: why did each planet develop with a distinct and very different composition? (CREDIT: CC BY-SA 4.0)
The formation of our solar system from a singular nebula raises an intriguing question: why did each planet develop with a distinct and very different composition? This diversity stems from a combination of factors, including the varying conditions within the protoplanetary disk, the processes of planetary differentiation, and the dynamic interactions among forming celestial bodies.
The Protoplanetary Disk and Planet Formation
Approximately 4.6 billion years ago, our solar system originated from a vast cloud of gas and dust known as the solar nebula. As this nebula collapsed under its own gravity, it formed a rotating disk around the nascent Sun, referred to as the protoplanetary disk. Within this disk, particles collided and coalesced, gradually building up into larger bodies called planetesimals, which eventually became planets.
The temperature gradient within the protoplanetary disk played a crucial role in determining the materials that could condense at various distances from the Sun.
Near the Sun, where temperatures were higher, only materials with high melting points, such as metals and silicates, could solidify. This led to the formation of the terrestrial planets—Mercury, Venus, Earth, and Mars—which are composed primarily of rocky substances.
Farther from the Sun, beyond the "frost line," temperatures were low enough for volatile compounds like water, methane, and ammonia to condense into ices. This allowed for the formation of the gas giants—Jupiter and Saturn—and the ice giants—Uranus and Neptune—which contain significant amounts of these ices and gases.
Planetary Differentiation
As planets formed and accumulated mass, their internal heat—generated from radioactive decay and the energy from accretion—caused them to partially or fully melt.
This melting initiated a process known as planetary differentiation, where denser materials, like iron and nickel, sank toward the center to form a core, while lighter materials rose to form a mantle and crust. This process resulted in layered structures within the planets and contributed to their compositional differences.
Related Stories
The solid Earth consists of distinct compositional layers: the dense, iron-rich metallic core; the less dense, magnesium-silicate-rich mantle; and the relatively thin, lightweight crust, primarily composed of aluminum, sodium, calcium, and potassium silicates. Above these layers, even lighter materials form the liquid hydrosphere and the nitrogen-rich gaseous atmosphere.
In general, lighter materials tend to rise through denser surroundings. For instance, low-density minerals like plagioclase can ascend, sometimes forming dome-like structures known as diapirs. On Earth, salt domes represent salt diapirs pushing upward through the crust.
Similarly, molten, low-density silicate rocks like granite frequently form diapirs in the upper crust. In subduction zones, hydrated, low-density serpentinite—formed by the alteration of mantle material—can also rise to the surface in this manner. Other substances exhibit similar behavior; for example, near the surface, mud volcanoes provide a low-temperature instance of this process.
Dynamic Interactions and Migration
The early solar system was a dynamic and turbulent environment, where planetary migration significantly influenced the formation of celestial bodies. Jupiter’s movements, in particular, played a crucial role in shaping the inner solar system by altering the distribution of planetesimals and shifting material that would eventually form the terrestrial planets.
The Grand Tack hypothesis suggests that Jupiter originally formed at a distance of 3.5 AU from the Sun before migrating inward to 1.5 AU. However, its course reversed when it became locked in an orbital resonance with Saturn, ultimately stopping near its present location at 5.2 AU. This reversal is compared to the maneuver of a sailboat tacking against the wind, hence the name "Grand Tack."
As Jupiter moved inward, it truncated the planetesimal disk at 1.0 AU, reducing the material available for planetary formation, particularly affecting Mars. During this process, Jupiter crossed the asteroid belt twice, first scattering asteroids outward and then driving them inward. This chaotic reshuffling resulted in the asteroid belt's current low mass and its mix of objects originating from both inside and outside Jupiter’s original orbit. Additionally, debris from planetesimal collisions in Jupiter’s path may have forced an early generation of planets to spiral into the Sun.
The Grand Tack hypothesis provides a compelling explanation for why Mars is smaller than expected and why the asteroid belt contains such a diverse range of objects. By modeling Jupiter’s early migration, astronomers gain insight into the intricate gravitational interactions that shaped the solar system as we see it today.
Influence of Stellar Chemistry
Recent studies have explored the relationship between a star's chemical composition and that of its planets. Research led by Vardan Adibekyan at the University of Porto examined the compositions of 22 rocky exoplanets with masses less than 10 Earths.
These exoplanets orbit 21 Sun-like stars. As expected, these observations revealed a clear link between the compositions of the stars and their planets. However, the relationship was not 1:1 as had been expected. Instead, the linear relationship between exoplanet iron and stellar iron has a slope of greater than four. In addition, the five iron-rich super Mercuries in the study appear as outliers when compared to the 17 super Earths.
The findings revealed that while there is a correlation between the chemical compositions of rocky planets and their host stars, the relationship is more complex than previously thought. This suggests that local conditions within the protoplanetary disk and the specifics of planetary formation processes play significant roles in determining planetary compositions.
Asteroid Evidence and Organic Compounds
Asteroids, often considered remnants from the early solar system, provide valuable insights into planetary formation. NASA's OSIRIS-REx mission returned samples from the asteroid Bennu, revealing a diverse array of organic compounds, including amino acids and nucleobases—the building blocks of life.
These findings support the idea that molecules carried on meteorites may have contributed to the emergence of life on Earth around 4 billion years ago. The analysis suggests that Bennu, a fragment of a larger asteroid, once harbored a wet and saline environment conducive to forming organic compounds, though it lacked conditions to develop primordial organisms.
Nicky Fox, associate administrator, Science Mission Directorate at NASA Headquarters in Washington wrote, “Asteroids provide a time capsule into our home planet’s history, and Bennu’s samples are pivotal in our understanding of what ingredients in our solar system existed before life started on Earth.”
Challenges to Existing Theories
Discoveries of exoplanets with unexpected characteristics have prompted reevaluations of existing planetary formation theories. For instance, the detection of a planet more than 13 times as massive as Earth orbiting the ultracool star LHS 3154 challenges traditional models, as the mass ratio of the planet to its host star is significantly higher than that of Earth and the Sun. Such findings suggest that planet formation processes may be more diverse than previously understood.
Another such discovery is the planet TIDYE-1b, identified by researchers at the University of North Carolina at Chapel Hill. Estimated to be just 3 million years old, TIDYE-1b formed in a fraction of the time previously believed necessary for planet formation, challenging the traditional view that planets require tens of millions of years to develop. This finding suggests that under certain conditions, planet formation can occur much more rapidly than current models predict.
Another challenge to existing theories comes from the discovery of two planets in close proximity to a hot Jupiter in the WASP-132 system. Traditionally, it was believed that the migration of hot Jupiters would destabilize the orbits of neighboring planets, leading to their ejection or destruction. However, this new research suggests that a more gradual migration within a protoplanetary disk may preserve the orbits of nearby planets, offering a new perspective on how these systems evolve.
Further complicating our understanding is the detection of free-floating planetary-mass objects, often referred to as "rogue planets." These objects, which do not orbit a star, challenge the traditional view that planets form exclusively within protoplanetary disks around stars. Their existence suggests alternative formation mechanisms, such as the direct collapse of gas and dust or ejection from developing planetary systems. The James Webb Space Telescope has recently provided detailed observations of such objects, offering new insights into their origins and challenging existing models of planet formation.
Additionally, the discovery of a "forbidden" planet orbiting a small star has raised questions about gas giant formation theories. Researchers from Carnegie Science identified a gas giant orbiting a low-mass star, a scenario previously thought unlikely due to insufficient material in the protoplanetary disk to form such a planet. This finding suggests that gas giant planets can form around a wider range of stellar types than previously believed, challenging existing models of planet formation.
These discoveries underscore the complexity of planetary formation and highlight the need for revised models that can accommodate the diverse range of planetary systems observed. As new observational technologies and methods continue to advance, our understanding of planet formation will undoubtedly evolve, potentially leading to new paradigms that better explain the rich variety of planetary systems in our universe.
The distinct compositions of the planets in our solar system are the result of a complex interplay of factors, including the temperature variations within the protoplanetary disk, processes of planetary differentiation, dynamic migrations, and the specific chemical environments in which they formed.
Ongoing research continues to uncover new insights, challenging existing theories and enhancing our understanding of the intricate processes that lead to the formation of diverse planetary systems.
Note: Materials provided above by The Brighter Side of News. Content may be edited for style and length.
Like these kind of feel good stories? Get The Brighter Side of News' newsletter.
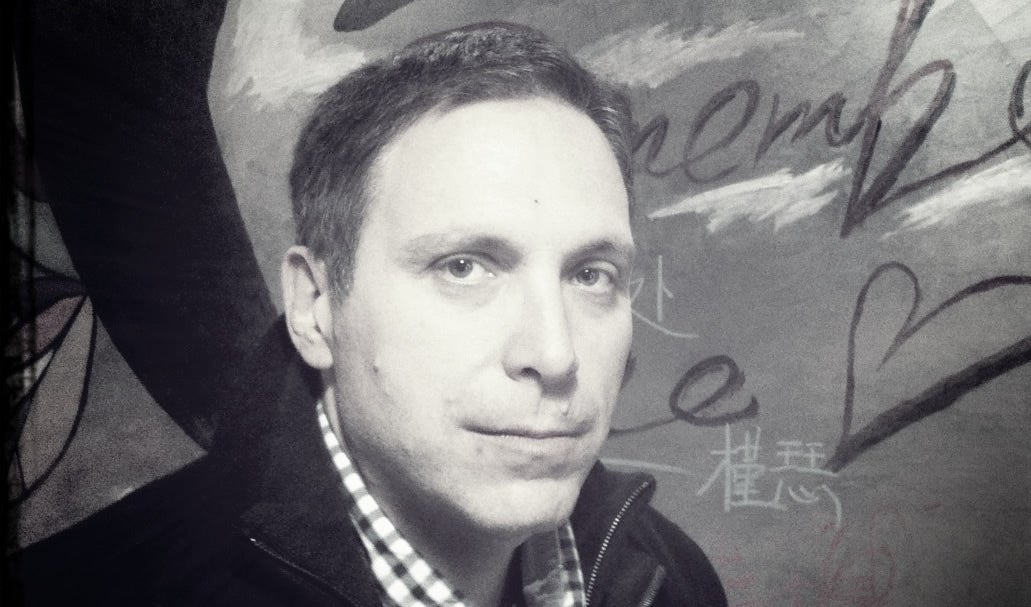
Joseph Shavit
Head Science News Writer | Communicating Innovation & Discovery
Based in Los Angeles, Joseph Shavit is an accomplished science journalist, head science news writer and co-founder at The Brighter Side of News, where he translates cutting-edge discoveries into compelling stories for a broad audience. With a strong background spanning science, business, product management, media leadership, and entrepreneurship, Joseph brings a unique perspective to science communication. His expertise allows him to uncover the intersection of technological advancements and market potential, shedding light on how groundbreaking research evolves into transformative products and industries.