First-ever thorium nuclear optical clock – the world’s most accurate timekeeper
A nuclear clock using thorium-229 could redefine timekeeping and test the laws of physics with record-breaking precision.
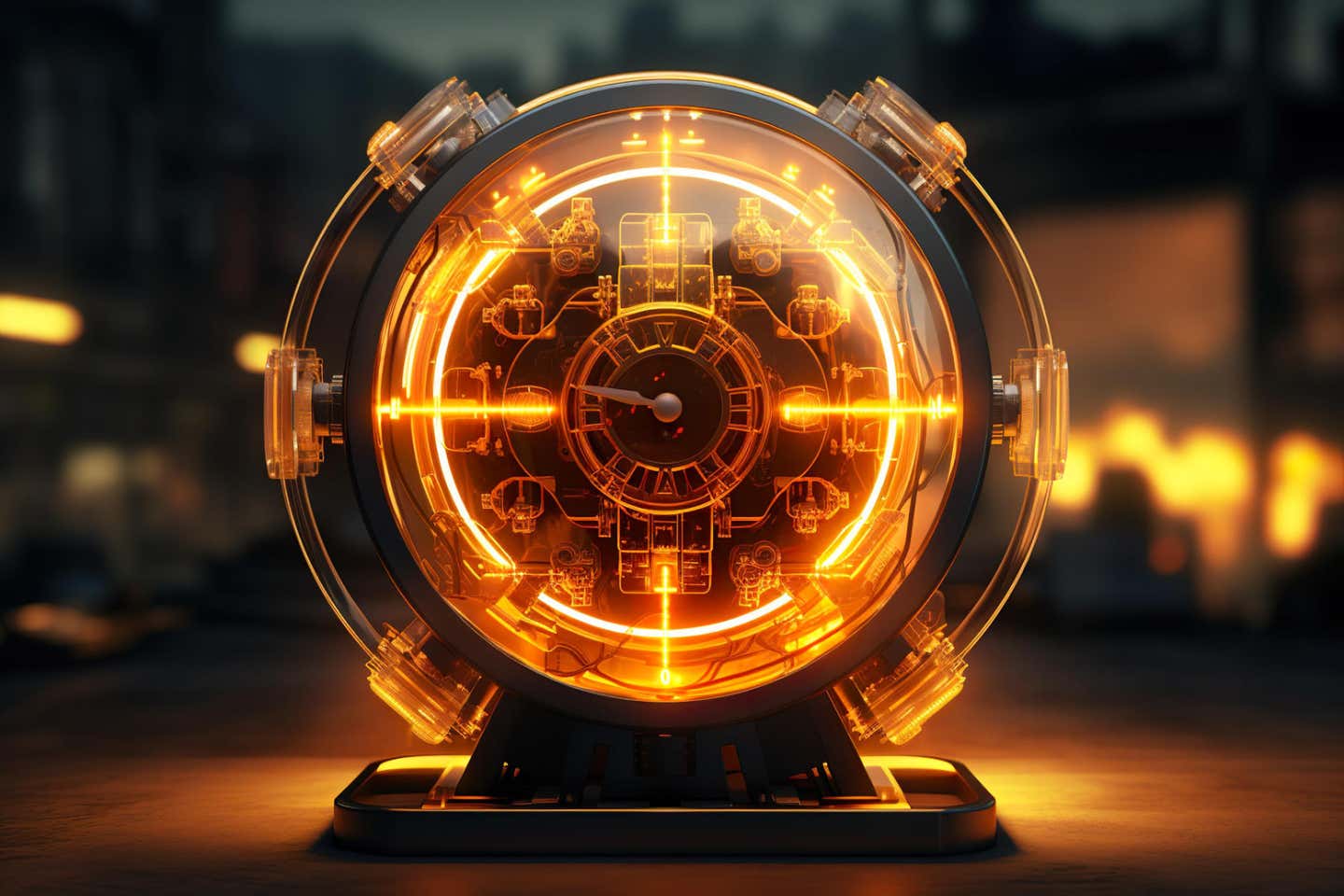
Graphic concept of a new kind of clock, powered by thorium-229, promises to redefine precision timekeeping and open new doors in physics research. (CREDIT: CC BY-SA 4.0)
Atomic clocks have long been the gold standard for measuring time and frequency. Among them, optical clocks—using atoms like strontium or aluminum—have reached staggering levels of accuracy, with relative uncertainties as low as one part in 10²⁰. But a new kind of clock, based not on electrons but on the nucleus of an atom, is on the horizon. This nuclear clock promises to push precision even further, opening up new frontiers in science.
A clock that ticks at the nuclear level
At the heart of this effort lies thorium-229, a rare isotope with a one-of-a-kind feature: it has a nuclear state that can be triggered using laser light. While most atomic clocks rely on changes in energy levels of electrons outside the nucleus, this isotope allows scientists to work with the quantum states inside the nucleus itself.
The first excited state of thorium-229 sits just 8.4 electron volts above its ground state. That’s low enough to be excited with a laser beam of around 148 nanometers—deep in the vacuum ultraviolet range. This is extremely rare, since nuclear energy levels are usually much higher and out of reach of current lasers. The ability to access a nuclear state with light makes thorium-229 a unique candidate for a nuclear optical clock.
This isn’t just a curiosity. A nuclear clock could be more precise than any clock ever built. That’s because nuclear states are less affected by magnetic fields, temperature changes, and electric noise than electron-based transitions. The nucleus is far smaller than an atom, and extranuclear electrons help shield it from environmental influences. These features make thorium-229 ideal for extreme precision, possibly reaching a relative uncertainty of one part in 10¹⁹ or better.
How the dream became a scientific goal
The idea of using thorium-229 in a nuclear clock dates back nearly 50 years. Researchers at the Idaho National Engineering Laboratory first spotted hints of a low-energy nuclear state in the 1970s. But for decades, the exact energy of that transition was unclear, and few believed it could be used in a practical clock.
By the early 2000s, the picture had begun to change. Re-analysis of old data confirmed that the nuclear excitation energy was indeed in the low-eV range. More recently, experiments have narrowed down the energy of the transition with higher accuracy, reaching terahertz precision.
Related Stories
Then came a major breakthrough. In 2024, scientists finally succeeded in using a laser to directly excite the nuclear transition of thorium-229. At Technische Universität Wien, researchers embedded thorium-229 atoms into calcium fluoride (CaF₂) crystals and exposed them to a 148-nanometer laser created at Germany’s Physikalisch-Technische Bundesanstalt. The light came from a four-wave mixing vacuum ultraviolet source, a difficult but powerful tool for exciting the nucleus.
Soon after, a team at UCLA did a similar experiment with lithium-strontium-aluminum fluoride crystals, again doped with thorium-229. Both studies managed to measure the nuclear transition frequency with an uncertainty of just one part in a million.
Precision leaps with advanced tools
That was just the beginning. In another leap forward, scientists at the Joint Institute for Laboratory Astrophysics (JILA) used a vacuum ultraviolet frequency comb to scan the transition. This tool is like a ruler made of laser light, with very fine and regular “teeth” that help measure light frequencies with extraordinary precision.
Using this method, they reduced uncertainty by six more orders of magnitude—down to the kilohertz level. That level of accuracy makes it possible to study subtle features of the nucleus, including its quadrupole splitting, a tiny effect that reveals internal structure.
Later, researchers at UCLA and JILA teamed up again. This time, they used thorium tetrafluoride (ThF₄) thin films instead of bulk crystals. While the measurements weren’t as precise—limited by the laser’s line width to the gigahertz level—they proved that a functional nuclear clock could be built with much smaller amounts of radioactive material.
These thin films drastically lower the amount of thorium needed, reducing safety concerns and material costs. It’s another step closer to a practical, real-world nuclear clock.
A rocky road: temperature, lasers, and supply issues
Even with these advances, big hurdles remain. One of the toughest problems is stability. In solid-state systems like crystals or thin films, the environment around the thorium nucleus affects its energy levels. Small changes in temperature can shift the nuclear frequency. To reach a precision of one part in 10¹⁸, scientists would need to keep the crystal temperature steady within 5 microkelvin. That’s nearly impossible outside of ideal lab conditions.
To get around this, researchers are exploring gas-phase thorium ions. In these systems, thorium atoms are ionized and trapped in vacuum chambers. With laser cooling, they can be brought to millikelvin temperatures and organized into crystal-like structures. These isolated systems are much less affected by outside noise and could provide long interaction times between ions and laser light. That makes them strong candidates for the next generation of ultra-precise nuclear clocks.
Still, there are other barriers. First, thorium-229 is extremely rare. Producing and purifying it is both expensive and technically difficult. The global supply is limited, and only small amounts are available for research.
Second, the laser needed to excite the transition—at 148 nanometers—has to be both powerful and ultra-precise. But building lasers that operate in this deep UV range is extremely difficult. Optical materials and components that work well at visible or near-infrared wavelengths often don’t perform at these short wavelengths. Creating a continuous-wave laser with a narrow line width at 148 nm remains an unsolved challenge.
Third, the nuclear transition doesn’t operate in isolation. It can interact with electron energy levels and surrounding electric fields. These interactions are still poorly understood, and they add complexity to designing a stable clock.
Finally, there's the issue of control. A working clock needs a closed-loop system. That means the clock must not only excite the nuclear state but also detect it, reset it, and repeat the cycle. This type of feedback loop hasn’t been achieved yet. Without it, creating a working prototype remains just out of reach.
A clock for more than just timekeeping
Even so, researchers remain hopeful. A working thorium-229 nuclear clock could go far beyond just keeping time. It could probe the very fabric of the universe.
With such extreme accuracy, a nuclear clock could detect tiny shifts in the values of fundamental constants—like the fine-structure constant, which controls the strength of electromagnetic interactions. If this constant changes over time or in different parts of space, a nuclear clock could spot it.
This could help test theories beyond the Standard Model of physics. It could offer clues about dark matter or other unexplained forces. A nuclear clock might even play a role in studying gravity at quantum scales.
In a recent article in National Science Review, Dr. Xin Tong and his team at the Chinese Academy of Sciences emphasized how close we are to unlocking these possibilities. They highlighted both the promise and the remaining technical hurdles that stand in the way.
The path forward will require new technology and greater understanding of the nucleus and its interactions. But the reward is clear: a tool that doesn’t just tell time better than any other, but one that could change how we understand space, time, and the universe itself.
Note: The article above provided above by The Brighter Side of News.
Like these kind of feel good stories? Get The Brighter Side of News' newsletter.
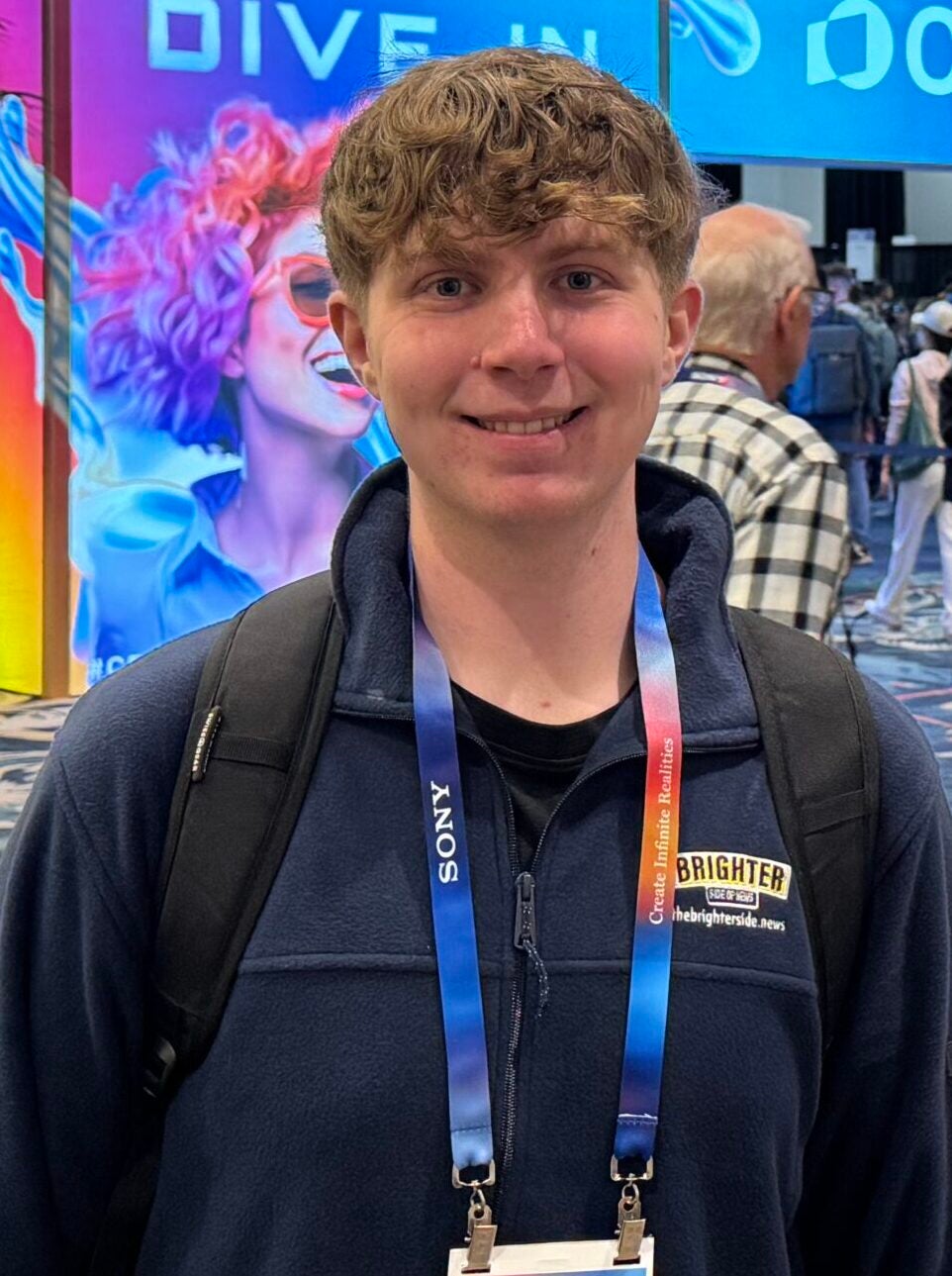
Joshua Shavit
Science & Technology Writer | AI and Robotics Reporter
Joshua Shavit is a Los Angeles-based science and technology writer with a passion for exploring the breakthroughs shaping the future. As a contributor to The Brighter Side of News, he focuses on positive and transformative advancements in AI, technology, physics, engineering, robotics and space science. Joshua is currently working towards a Bachelor of Science in Business Administration at the University of California, Berkeley. He combines his academic background with a talent for storytelling, making complex scientific discoveries engaging and accessible. His work highlights the innovators behind the ideas, bringing readers closer to the people driving progress.