Danish student makes groundbreaking black hole discovery
Discover the groundbreaking insights into gravitational lensing and black holes, unraveling elegant mathematics and revealing cosmic mysteries.
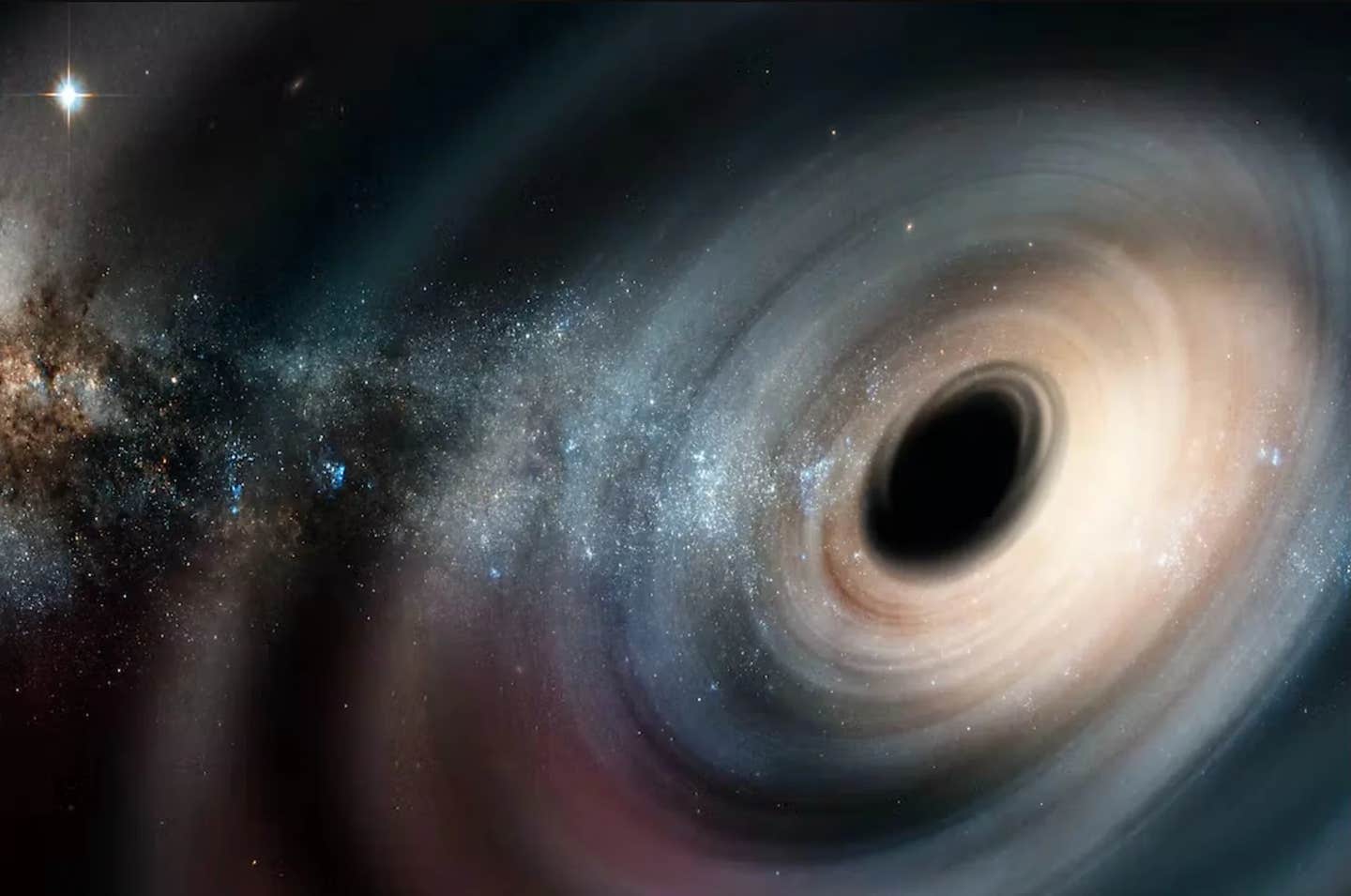
Light bends around black holes, creating infinite repetitions of the universe’s image. (CREDIT: interstellar.wiki/CC BY-NC License).
Black holes have long captivated scientists and the public, embodying the mysteries of the universe. These celestial phenomena warp space-time so drastically that light itself bends around them, offering insights into the nature of gravity and the cosmos. At their event horizons, not even light can escape their pull, making black holes one of the most enigmatic features of the universe.
Light interacts with black holes in intriguing ways. While photons passing too close to the event horizon are inevitably swallowed, those farther away may escape, often after completing several orbits around the black hole.
This process occurs near a critical boundary called the photon-sphere. Here, light follows unstable circular paths, spiraling either outward to infinity or inward toward the event horizon.
The closer a photon approaches the photon-sphere, the more orbits it completes before escaping. Observers see light from every point in space orbit the black hole multiple times, creating an intricate visual effect.
From afar, this manifests as infinite repetitions of the universe’s image near the edges of a black hole's shadow. Each orbit brings light closer to the black hole’s edge, resulting in a strikingly detailed mapping of the cosmos.
This phenomenon has fascinated researchers for decades, particularly for its mathematical precision. The deflection angle of light in these orbits diverges logarithmically as it nears the event horizon. Recent advances have refined this understanding, offering not only new insights into the trajectories of photons but also innovative ways to analyze the exponential growth of small perturbations in these paths.
A Fresh Analytical Perspective
A groundbreaking approach has reframed this phenomenon by analyzing light’s trajectory near black holes using second-order differential equations. This methodology examines the stability of photon paths, revealing that deviations from the photon-sphere grow exponentially.
Related Stories
Each additional orbit forms an exponentially thinner ring around the black hole, creating a visual cascade of images. This insight not only deepens our understanding of gravitational lensing but also highlights the dueling exponential forces shaping these trajectories.
The method's flexibility allows it to be applied to various types of black holes, including rotating ones. This extension has revealed how black hole spin influences the spatial arrangement of photon paths.
For example, in spinning black holes, prograde (forward-moving) images become more closely spaced, while retrograde (backward-moving) ones spread out. This discovery opens new avenues for exploring black hole dynamics.
The Mathematics Behind the Phenomenon
While gravitational lensing has been a well-studied concept, a recent development has shed new light on the phenomenon’s underlying mathematics.
Albert Sneppen, a student researcher at the Niels Bohr Institute, uncovered an elegant proof explaining why repeated images near a black hole are separated by a specific factor.
Published in Scientific Reports, his work reveals the physical and mathematical intuition behind this factor, deepening our grasp of gravitational lensing.
Sneppen’s work demonstrates that each successive image requires observing 500 times closer to the black hole than the previous one. This factor, deeply rooted in the fundamental nature of gravity, provides a unique way to test Einstein’s theories.
As Sneppen noted, “There is something fantastically beautiful in now understanding why the images repeat themselves in such an elegant way.”
Insights into Rotating Black Holes
The implications of Sneppen’s findings extend beyond static black holes. All black holes rotate to varying degrees, and their spin significantly alters the gravitational lensing process.
In rapidly spinning black holes, the separation factor for successive images drops significantly. Instead of needing to look 500 times closer for each image, the factor might shrink to 50, 5, or even 2 times closer. This makes the phenomenon more observable and offers exciting possibilities for future research.
When black holes rotate, additional space is created for light paths, making extra images more accessible. This opens the door to empirical confirmation of theoretical predictions, providing insights into not only black holes but also the galaxies that lie behind them.
Gravitational lensing is not just a theoretical curiosity—it has practical implications. Light’s travel time increases with each orbit, introducing significant delays in successive images.
For instance, if a supernova occurs in a galaxy behind a black hole, observers could witness the explosion multiple times, each view separated by measurable delays. This effect provides a powerful tool for studying cosmic events and testing gravitational theories.
The ability to map repeating images near black holes offers a unique opportunity to explore the universe. Each successive image carries new information about the galaxies behind the black hole, enriching our understanding of cosmic structures and phenomena. These insights hold the potential to revolutionize fields ranging from astrophysics to fundamental physics.
The elegant mathematical framework introduced by Sneppen and others promises to inspire a new generation of scientific inquiry. By refining our understanding of light’s behavior near black holes, researchers can test the boundaries of Einstein’s theories, explore the effects of black hole spin, and unlock the secrets of distant galaxies.
As observational technologies advance, the potential for discovering new phenomena through gravitational lensing grows exponentially.
Black holes may be the ultimate enigmas of the universe, but their study continues to illuminate some of its deepest mysteries. With each orbit of light and each repetition of an image, we move closer to unraveling the fundamental principles that govern space, time, and gravity.
Note: Materials provided above by The Brighter Side of News. Content may be edited for style and length.
Like these kind of feel good stories? Get The Brighter Side of News' newsletter.
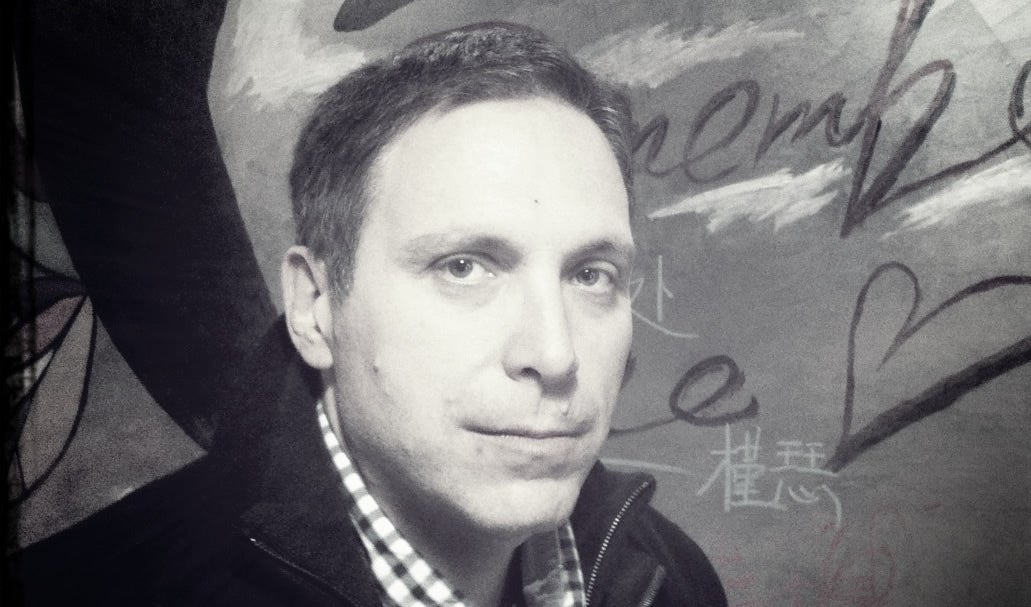
Joseph Shavit
Head Science News Writer | Communicating Innovation & Discovery
Based in Los Angeles, Joseph Shavit is an accomplished science journalist, head science news writer and co-founder at The Brighter Side of News, where he translates cutting-edge discoveries into compelling stories for a broad audience. With a strong background spanning science, business, product management, media leadership, and entrepreneurship, Joseph brings a unique perspective to science communication. His expertise allows him to uncover the intersection of technological advancements and market potential, shedding light on how groundbreaking research evolves into transformative products and industries.