Compact “gene scissor” revolutionizes genetic engineering and editing
Scientists have engineered TnpB, a smaller, more efficient alternative to CRISPR-Cas9, showing potential for treating genetic diseases like hypercholesterolemia.
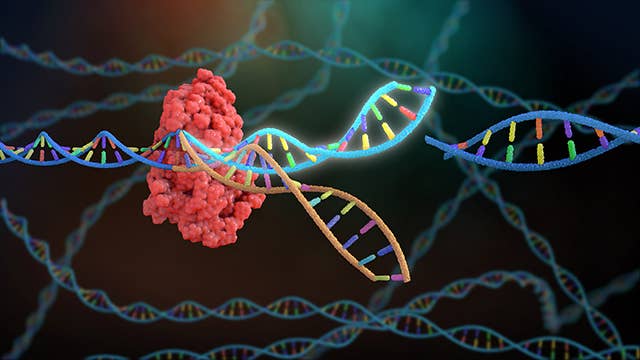
The TnpB protein is much smaller than the well-known CRISPR-Cas gene scissors and thus easier to transport into cells. (CREDIT: iStock/Nathan Devery)
CRISPR-Cas systems, originally a natural defense mechanism in bacteria, have become a groundbreaking tool for genetic engineering in medicine. These “gene scissors” can precisely edit specific locations in our DNA, correcting disease-causing mutations.
However, the large size of the proteins used in CRISPR systems, like Cas9 and Cas12, can complicate the delivery of the tools into the right cells in the body. Researchers have been working to overcome this challenge by turning to smaller protein alternatives, such as TnpB, which have the potential to be more efficient and easier to deliver.
Recently, scientists have made important strides in improving the efficiency of TnpB for gene editing. TnpB is an evolutionary predecessor of Cas12, and its compact size has sparked interest in using it as a tool for genome editing.
Unfortunately, TnpB was initially less effective, but a team led by Gerald Schwank from the University of Zurich, working with colleagues from ETH Zurich, has successfully engineered a version of TnpB that boosts its efficiency by 4.4 times.
According to Schwank, "By engineering the small but powerful protein TnpB, we were able to design a variant that shows a 4.4-fold increase in efficiency of modifying DNA – making it more effective as a gene editing tool.”
TnpB proteins are found in a wide range of bacteria and archaea. The specific TnpB studied by the researchers comes from Deinococcus radiodurans, a microorganism known for its incredible resilience to extreme conditions like radiation, dehydration, and cold.
Related Stories
Despite this microbe’s impressive survival abilities, the original TnpB protein from Deinococcus demonstrated low efficiency and limited ability to target DNA sequences accurately, making it less than ideal for genome editing in human cells. However, recent efforts to modify the protein have improved its effectiveness.
The research team made two key modifications to the TnpB protein to increase its efficiency. First, they improved its ability to move into the nucleus of mammalian cells, where DNA is located. Second, they enhanced the protein’s ability to recognize and bind to a broader range of DNA sequences.
These changes made it much more effective for gene editing in mammalian cells. “The trick was to modify the tool in two ways: first, so that it more efficiently goes to the nucleus where the genomic DNA is located, and second, so that it also targets alternative genome sequences,” explained Kim Marquart, a PhD student working in Schwank’s lab and the first author of the study.
To further refine the process, the researchers collaborated with Professor Michael Krauthammer’s team at the University of Zurich to develop an artificial intelligence model. This AI model can predict the efficiency of TnpB editing at any given DNA target site.
The team tested the protein at 10,211 different target sites to gather data for the AI model. Marquart said, “Our model can predict how well TnpB will work in different scenarios, making it easier and faster to design successful gene editing experiments. Using these predictions, we achieved up to 75.3% efficiency in mouse livers and 65.9% in mouse brains.”
One of the major advantages of using TnpB as a gene-editing tool is its smaller size compared to traditional CRISPR-Cas systems. The research team successfully packaged the TnpB system into a single viral particle, allowing it to be delivered more efficiently into the cells of mice.
“For the animal experiments, we were able to use clinically viable Adeno-associated viral vectors to efficiently transport the tools into mouse cells. Due to its small size, the TnpB gene editing system can be packaged into a single virus particle,” Marquart stated. This is a significant improvement over CRISPR-Cas9, which requires multiple virus particles, often resulting in higher vector doses and added complications.
In their most recent experiments, the researchers focused on familial hypercholesterolemia, a genetic disorder affecting around 31 million people globally. This disease causes lifelong high cholesterol levels, which can lead to early-onset cardiovascular diseases like atherosclerosis.
Using TnpB, the team edited a gene responsible for regulating cholesterol in mice. The results were remarkable, with cholesterol levels in treated mice reduced by nearly 80%. Schwank noted, “We were able to edit a gene that regulates cholesterol levels, thereby reducing the cholesterol in treated mice by nearly 80%. The goal is to develop similar gene editing strategies in humans in order to treat patients suffering from hypercholesterolemia.”
This promising research opens the door to potential gene-editing therapies for genetic disorders in humans. The enhanced efficiency and smaller size of TnpB make it a powerful tool for future medical applications, with the possibility of treating diseases that are currently incurable.
By continuing to refine this technology and its delivery methods, scientists may one day use TnpB to correct genetic defects in humans, offering new hope for patients with conditions like familial hypercholesterolemia.
Note: Materials provided above by The Brighter Side of News. Content may be edited for style and length.
Like these kind of feel good stories? Get The Brighter Side of News' newsletter.