Breakthrough quantum computer unlocks hidden world of elementary particles
Qudit-based quantum computers simulate 2D quantum electrodynamics, revealing magnetic fields and particle interactions in new detail.
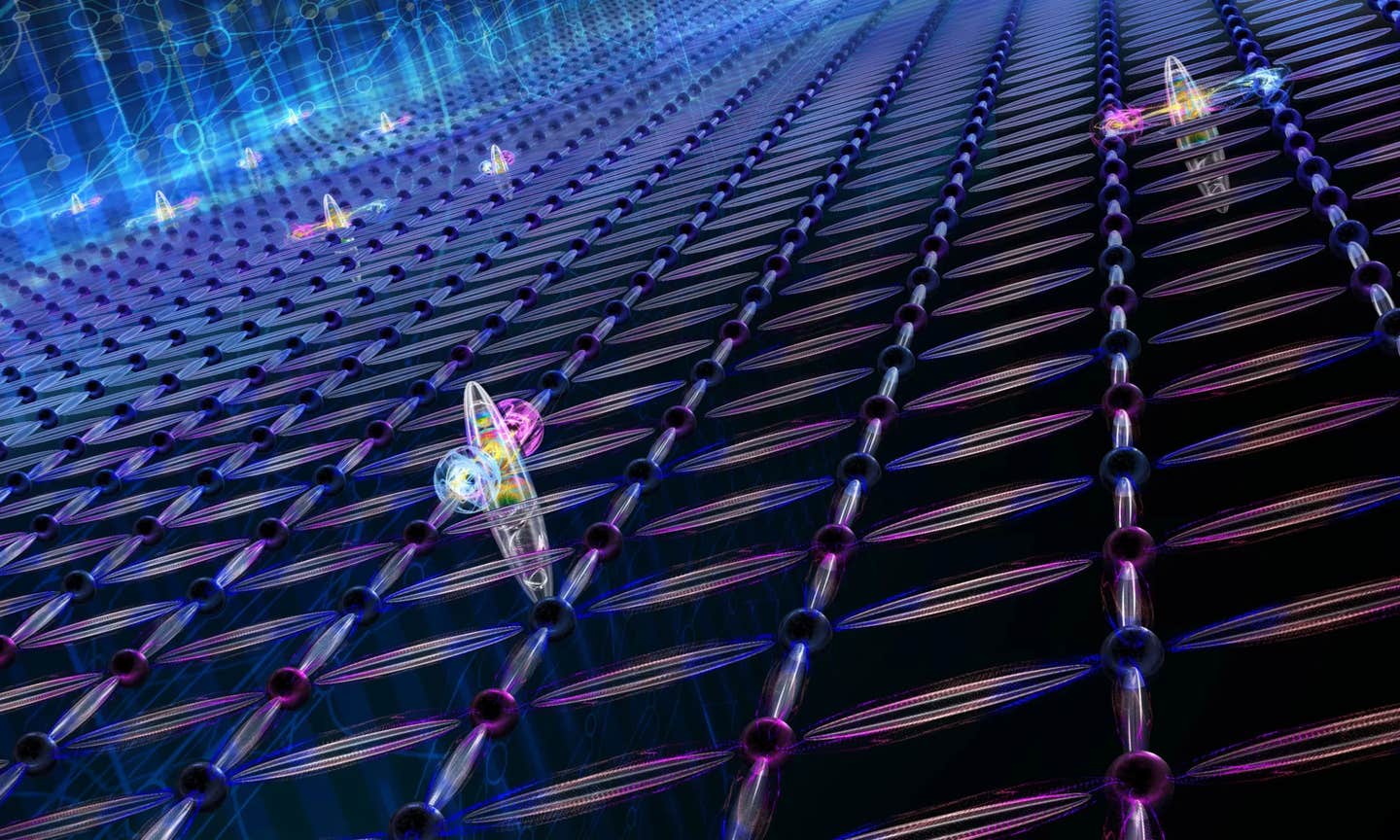
Simulation of the formation of particle-antiparticle pairs in two spatial dimensions. (CREDIT: Harald Ritsch)
In today’s world of science, understanding the building blocks of nature means diving deep into the strange, complex world of quantum physics. At the heart of this effort lies the study of particle interactions and the invisible forces that guide them—forces explained by what scientists call gauge theories. These theories describe how particles interact by exchanging force-carrying particles, forming the basis of our best understanding of matter through the Standard Model of particle physics.
But working with gauge theories on a computer is no easy task. That’s because the rules that govern these particles don’t follow everyday logic. They’re ruled by quantum mechanics, where things can exist in many states at once, particles pop in and out of existence, and everything is connected through entanglement. These complexities make it extremely hard to simulate quantum systems using traditional, or even most modern, supercomputers.
Going Beyond Binary
Computers—whether classical or quantum—usually speak in binary. A regular computer processes bits that are either a 0 or a 1. Quantum computers use qubits, which can be both 0 and 1 at once thanks to a phenomenon called superposition. That alone gives them a big advantage when dealing with quantum problems. But there’s still a limit.
Some of the most difficult physics problems, like simulating real-time dynamics in quantum field theories or understanding dense forms of matter, simply go beyond what even powerful quantum computers using qubits can handle. These problems involve sign issues, where numerical simulations become too unstable or unreliable, making classical techniques nearly impossible.
Now, a new kind of quantum computer is helping scientists break through those limits—by going beyond qubits.
The Rise of the Qudit
This innovation centers around something called a qudit. Instead of storing just two possible values (like a qubit), a qudit can store multiple energy levels—three, four, five, or more. It’s like trading a simple light switch for a dimmer with many settings.
This added flexibility gives quantum computers an edge when simulating gauge fields, which naturally exist in higher dimensions. These fields represent things like electric and magnetic forces, which don’t fit neatly into binary systems. By using qudits, scientists can mimic these complicated fields much more directly and efficiently.
Related Stories
In a recent experiment, a team from the University of Innsbruck in Austria, led by physicist Martin Ringbauer, teamed up with a theory group in Canada headed by Christine Muschik at the University of Waterloo. Together, they performed the first quantum simulation of a gauge theory with both particles and fields in two spatial dimensions—something never before achieved.
A Key Breakthrough in Quantum Electrodynamics
The team’s focus was on quantum electrodynamics in two dimensions (2D-QED). This is a simpler cousin of the theory that describes how light and matter interact in the real world. Even though it’s only in two dimensions, it still captures many essential parts of particle physics, like the interaction between particles and the electromagnetic field.
In the experiment, they created a simplified version of space—a plaquette, or a square-shaped piece of a lattice that mimics the fabric of space. On this small patch, they simulated how particles and gauge fields behave using a qudit quantum processor made from trapped ions. Each ion could represent more than two states, allowing the simulation to include more detail while using fewer resources.
This was more than a technical trick. It let the team observe magnetic fields between particles, something you can’t see in simpler, one-dimensional simulations. As Michael Meth, the study’s lead author, explained, “Our approach enables a natural representation of the quantum fields, which makes the computations much more efficient.”
In previous work, the same group had already shown how particles and antiparticles could be created in a quantum simulation. But that earlier demonstration only allowed particles to move in a line. Now, they’ve moved into true two-dimensional space, which allows for much richer and more realistic physics. “Removing this restriction is a critical step to using quantum computers to understand fundamental particle interactions,” said Muschik.
Smaller, Smarter, and More Scalable
One of the most exciting parts of this breakthrough is how efficient it is. Using high-fidelity qudit gates—essentially the rules that let qudits interact—they were able to drastically reduce the number of steps needed in the quantum circuit. That’s important because today’s quantum hardware is still fragile. The fewer operations needed, the more reliable the simulation.
In fact, for their basic simulation, the team saw an order of magnitude improvement in the complexity of their circuits compared to using qubits. That kind of leap opens up new opportunities for running real physics problems on near-term quantum hardware—not decades from now, but soon.
Controlling the dimension of the gauge fields was another key success. The team showed how adjusting the number of levels in a qudit could influence the simulation’s accuracy. This is a powerful tool for balancing performance with the physical detail of the model.
And because qudits can better reflect the high-dimensional nature of gauge fields, they help avoid the problem of “truncation” that occurs when cutting off parts of the physics to make them fit into simpler computational models.
The Road Ahead: Toward the Strong Force and Beyond
The significance of this work goes far beyond a single simulation. It marks a turning point in how scientists can explore particle physics using quantum computers.
Gauge theories, including QED, are part of the broader Standard Model, which also includes the strong nuclear force—the force that holds atomic nuclei together. This force remains one of the least understood areas in physics. But with just a few more qudits, the research team says their methods could be extended to cover three-dimensional models and even the strong force itself.
That could lead to answers for questions that have remained open for decades. How do particles behave under extreme conditions, like those found inside neutron stars? Can we simulate the early universe and understand how matter formed in the first moments after the Big Bang?
“Quantum computers offer a unique scientific opportunity for advancing the frontier of gauge theory simulations,” the researchers wrote. Unlike classical methods, quantum simulations are not disrupted by sign problems, making them ideal for exploring these challenging areas.
“This brings us an important step closer to studying nature,” said Ringbauer. “We are excited about the potential of quantum computers to contribute to the study of these fascinating questions.”
From Theoretical Dream to Physical Reality
Though still in its early days, this research proves that quantum computing—especially with qudits—can tackle real physics problems. It transforms what was once a theoretical dream into something testable and observable. The hope is that, as hardware improves and more qudits become available, larger and more complex simulations will unlock answers to some of science’s deepest mysteries.
By blending cutting-edge hardware with smart algorithms, researchers are setting the stage for a new kind of scientific discovery. The next chapter in understanding the universe might not come from building bigger particle accelerators, but from building better quantum computers—ones that don’t just compute with zeros and ones, but with something far more powerful.
Study findings are available in the journal Nature Physics.
Note: The article above provided above by The Brighter Side of News.
Like these kind of feel good stories? Get The Brighter Side of News' newsletter.
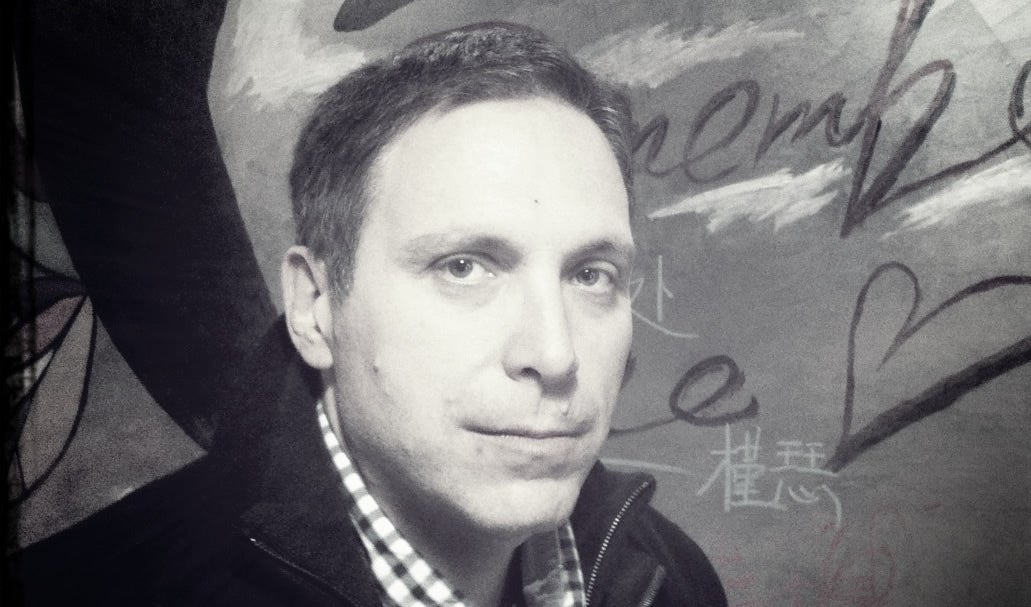
Joseph Shavit
Head Science News Writer | Communicating Innovation & Discovery
Based in Los Angeles, Joseph Shavit is an accomplished science journalist, head science news writer and co-founder at The Brighter Side of News, where he translates cutting-edge discoveries into compelling stories for a broad audience. With a strong background spanning science, business, product management, media leadership, and entrepreneurship, Joseph brings a unique perspective to science communication. His expertise allows him to uncover the intersection of technological advancements and market potential, shedding light on how groundbreaking research evolves into transformative products and industries.