Black hole pairs reveal hidden new forces and unseen particles
Scientists use gravitational waves to detect hidden ultralight particles around black holes, potentially solving physics’ biggest mysteries.
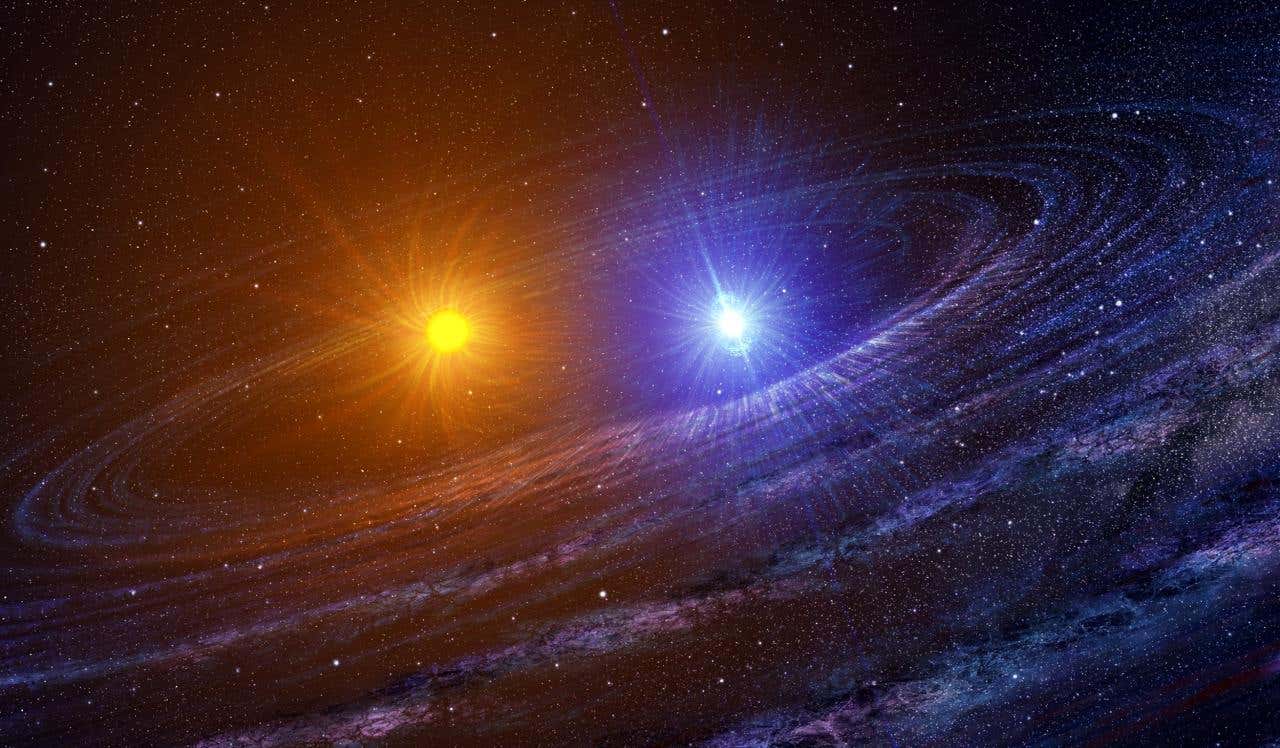
Physicists believe gravitational waves from merging black holes could uncover hidden ultralight particles. (CREDIT: Casey Reed, NASA)
When two black holes circle each other, their movements produce gravitational waves. These waves are ripples in space itself, carrying detailed clues about the objects that made them. Future detectors, such as LISA, aim to capture these signals, offering a new way to study hidden forces and unseen particles.
Recently, physicists have proposed that gravitational waves might help discover elusive particles known as ultralight bosons. These particles, much lighter than those detected in laboratories, could solve major puzzles in physics, including the mystery of dark matter.
Black holes might hold the key to finding these particles. When a black hole spins fast, it can shed mass and create a surrounding cloud of bosons through a unique process called superradiance. Scientists compare this to how electrons orbit a proton, calling it a "gravitational atom."
What is Superradiance
Superradiance is a quantum phenomenon where a group of excited atoms, instead of emitting light independently, release their energy collectively in a burst that is much more intense and faster than expected. This synchronized emission results from the quantum coherence between the atoms, allowing them to act as a single, unified system.
The concept was first postulated in 1954 by American physicist Robert H. Dicke, who introduced the idea while studying radiation emitted by ensembles of atoms. He theorized that under certain conditions, atoms within a small volume could become entangled in such a way that their emitted photons would interfere constructively, leading to an intense, directional beam of radiation.
Experimental confirmation of superradiance began to emerge in the 1970s, particularly in systems of dense atomic gases and later in solid-state systems like quantum dots and superconducting circuits.
Over the decades, superradiance has evolved from a theoretical curiosity to a robust area of research, with applications ranging from laser physics to quantum computing and even astrophysics.
At its core, superradiance showcases how collective quantum behavior can produce effects far beyond what classical physics would predict. It highlights the power of coherence and entanglement—two cornerstones of quantum theory—and their potential to revolutionize our understanding of light, matter, and energy transfer on both microscopic and cosmic scales.
A Cloud Born from Black Holes
In recent years, physicists have also explored “black hole superradiance,” a theoretical process in which rotating black holes could transfer energy to surrounding fields, potentially explaining dark matter candidates like axions.
Superradiance happens naturally, requiring no pre-existing particles. Instead, it taps into the black hole's spinning energy, forming a boson cloud around it. The efficiency of this process depends on a special relationship between the boson's mass and the black hole’s size. For boson clouds to form around real black holes, these particles must be incredibly light—far lighter than anything detected so far.
Ultralight bosons, proposed by different areas of physics, could be linked to dark matter, a mysterious substance that makes up most of the universe's mass but is invisible to current instruments. Understanding boson clouds might shed light on this great cosmic mystery.
Related Stories
Resonances and the Secrets of Black Hole Clouds
Physicists have been closely examining how a pair of orbiting black holes interacts with these boson clouds. They found that two important effects shape this interaction: ionization and resonances.
Ionization is similar to an atom losing electrons, where part of the cloud gets ejected as the black holes approach each other. Resonances are more subtle, occurring earlier and at greater distances. They happen when the orbiting black hole affects the cloud even from far away, causing the boson cloud to shift energy levels, much like electrons jumping between orbits in an atom.
“These resonant transitions are key,” explains physicist Giovanni Maria Tomaselli from the University of Amsterdam (UvA). “They can significantly alter the system, changing the shape and alignment of the orbit.”
This detailed interplay affects the gravitational waves emitted during the final stages of the black holes' merger. By analyzing these waves, scientists can uncover subtle signals hinting at the cloud’s existence and the nature of the bosons within it.
Tracking the History of a Cosmic Dance
Scientists emphasize that understanding the early history of a black hole binary system is critical. Before gravitational waves enter a detector's frequency range, the orbiting black hole can already influence the boson cloud. This interaction sets the stage for later events.
As black holes spiral closer, environmental effects, like resonances and ionization, accumulate over millions of orbits. Each orbit slightly changes the gravitational wave patterns, creating a detailed record of the cloud’s evolution.
Recently, researchers took a significant step forward, combining previous studies to track these complex interactions from start to finish. Physicist Gianfranco Bertone, also from the University of Amsterdam, notes, “Our latest findings help us understand exactly how these clouds behave during the entire merger process.”
Two Outcomes—Each Revealing Important Clues
This comprehensive study identified two distinct outcomes, each offering exciting opportunities for discovery. The result depends strongly on how the black holes and their boson clouds rotate.
If the black hole and the boson cloud initially spin in opposite directions, the cloud stays intact. Ionization happens later, clearly marking gravitational waves. Detecting these signs would be direct evidence of ultralight bosons. According to Bertone, “This case provides a strong, unmistakable signal. It would be like a fingerprint left behind.”
But if rotations align differently, resonant transitions break apart the boson cloud much earlier. This destruction imprints unique patterns on the binary’s orbit, changing its shape and orientation in measurable ways. In this scenario, scientists wouldn't detect the cloud directly. Instead, gravitational waves would show distinct orbits, signaling that a cloud once existed but vanished.
Both scenarios provide strong evidence for ultralight bosons. Tomaselli explains, “Whether the cloud survives or not, the gravitational waves we detect will tell us something important about these elusive particles.”
Future Observations May Solve Physics Mysteries
Upcoming gravitational wave detectors will soon search for these subtle signals. Instruments like LISA will precisely measure gravitational waves at frequencies sensitive enough to detect these small effects. Scientists believe that by carefully analyzing gravitational wave data, they'll confirm or rule out the existence of ultralight bosons.
If detected, these particles would solve several mysteries simultaneously. They could explain dark matter, help scientists understand fundamental physics, and even provide clues to longstanding problems like the strong CP issue in quantum physics.
Thomas Spieksma, who contributed to this groundbreaking research, adds, “We’re at a turning point. Gravitational waves might open a completely new chapter in physics.”
Why This Research Matters
This research connects astrophysics, particle physics, and cosmology in a unique way. Studying gravitational waves isn't just about black holes—it's about understanding the very building blocks of the universe. Boson clouds offer an unprecedented laboratory to test theories that were previously impossible to verify.
By identifying clear, observable signatures in gravitational waves, scientists have set the stage for major discoveries. The potential findings go beyond theoretical interest, promising to reshape our understanding of reality itself.
As Tomaselli says, “We're not just looking at gravitational waves. We're looking into the fundamental nature of our universe.”
Research findings are available in the journal Physical Review Letters.
Note: The article above provided above by The Brighter Side of News.
Like these kind of feel good stories? Get The Brighter Side of News' newsletter.
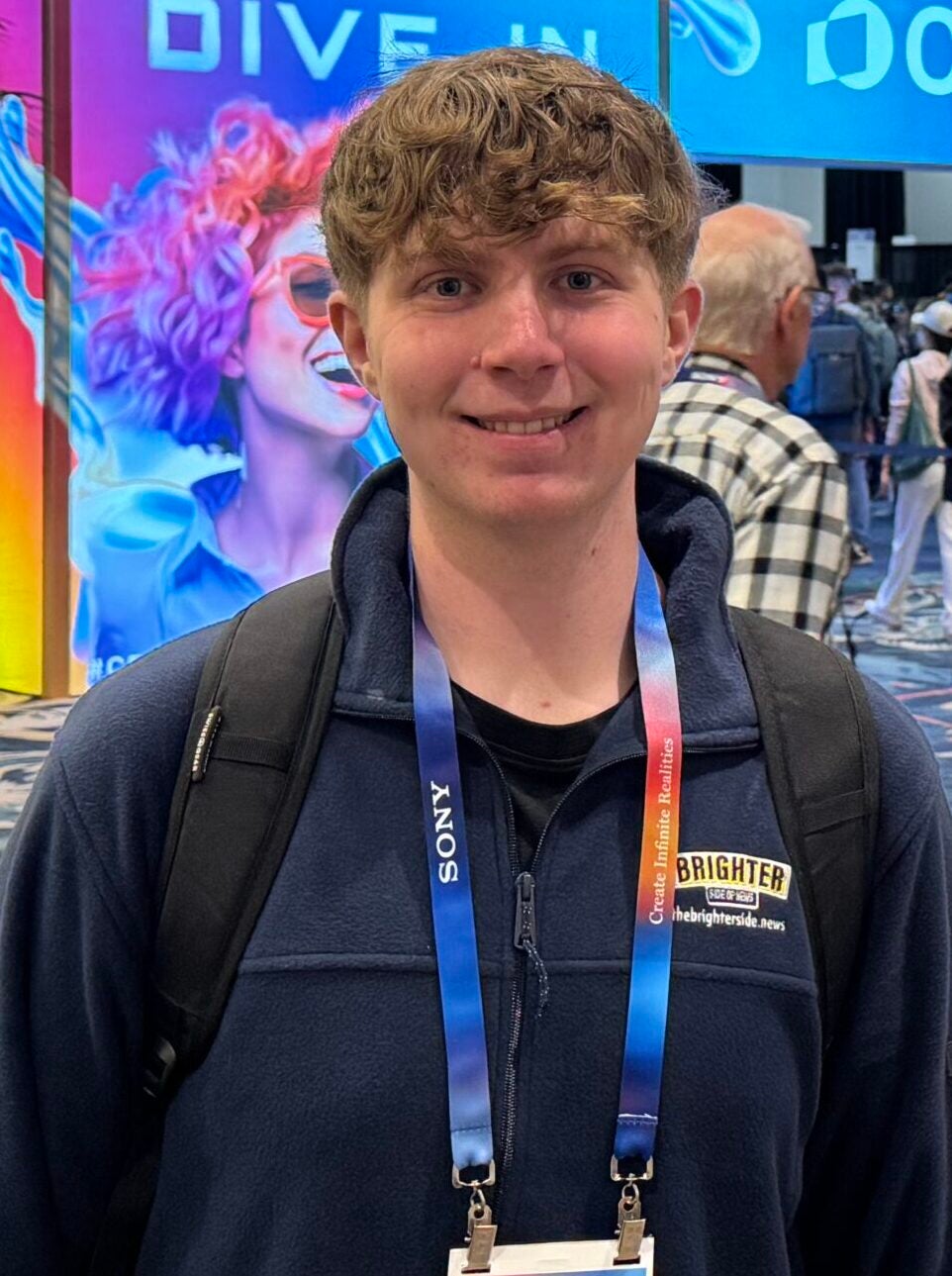
Joshua Shavit
Science & Technology Writer | AI and Robotics Reporter
Joshua Shavit is a Los Angeles-based science and technology writer with a passion for exploring the breakthroughs shaping the future. As a contributor to The Brighter Side of News, he focuses on positive and transformative advancements in AI, technology, physics, engineering, robotics and space science. Joshua is currently working towards a Bachelor of Science in Business Administration at the University of California, Berkeley. He combines his academic background with a talent for storytelling, making complex scientific discoveries engaging and accessible. His work highlights the innovators behind the ideas, bringing readers closer to the people driving progress.