Bizarre form of ice only melts at extremely hot temperatures
Inside planets, extreme pressures and temperatures transform familiar materials into strange and exotic forms.
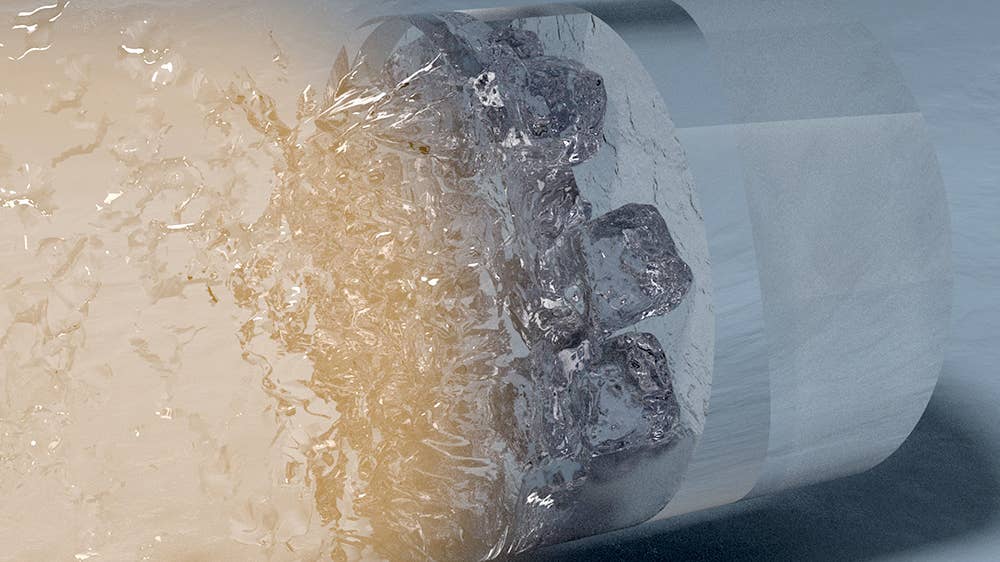
[Oct. 16, 2023: Staff Writer, The Brighter Side of News]
Artist rendering of Superionic ice. (CREDIT: Creative Commons)
Inside planets, extreme pressures and temperatures transform familiar materials into strange and exotic forms.
Within Earth's solid inner core, iron atoms dance, while in the depths of the gas giants Uranus and Neptune, a peculiar form of water exists—superionic ice. It's a perplexing substance: solid and liquid simultaneously, hot, dark, and heavy. Imagine ice that's both solid and flowing, simultaneously. Sound impossible? Well, the universe begs to differ.
Journey of Discovery: From Theoretical Ice to Lab Creation
This story began decades ago. In 1988, physicists prophesied a bizarre form of water that remained elusive until 2017. That year, scientists succeeded in recreating this exotic ice in lab settings, confirming its existence and crystalline structure a year later.
But the tale of superionic ice didn't end there.
Related Stories
Researchers from various esteemed American universities and the renowned Stanford Linear Accelerator Center laboratory in California (SLAC) unveiled a groundbreaking discovery just last year: a new phase of superionic ice.
This discovery unravels the long-standing mystery surrounding the peculiar magnetic fields of Uranus and Neptune, with their multiple poles and unpredictable orientations.
The Enigmatic Nature of Superionic Ice
For many of us, water is straightforward. It's a simple molecule—two hydrogens and an oxygen—that assumes a fixed form upon freezing. However, when water meets the unimaginable pressures, about 2 million times that of Earth's atmosphere, and the scorching temperatures akin to the Sun's surface within planets like Uranus and Neptune, it morphs into the mystifying superionic ice.
Neptune interior with multiple superionic layers. Ice giant interiors, like Neptune, have different layers of superionic ice. The molecular envelope of He, H2 and H2O gas is largely insulating and the convecting ionic liquid layer may have ionic conductivities of a few to 100 (Ω cm)−1. (CREDIT: Nature)
Reaffirming the 1988 prediction, scientists in 2019 confirmed a unique structure for superionic ice. Here, oxygen atoms create a solid cubic lattice, trapping them in place. Contrarily, the ionized hydrogen atoms run free, moving seamlessly through the lattice, much like electrons traversing metals.
This peculiar structure grants superionic ice its conductive properties, allowing it to remain solid even at sweltering temperatures.
Experimental configuration of the XFEL probe and optical laser pump. The shock solidification behavior of water is captured in a Debye–Scherrer geometry. Inset: Schematic of target package with cut away side-view of the water layer. (CREDIT: Nature)
Discovering Ice XIX: The Experiment
In the latest study spearheading our understanding of superionic ice, physicist Arianna Gleason of Stanford University, along with her team, subjected water to conditions mimicking the interiors of Neptune-like exoplanets. Using powerful lasers, they targeted thin water layers trapped between diamonds, producing shockwaves that amplified the pressure to a staggering 200 GPa, or 2 million atmospheres. These conditions simultaneously raised temperatures to around 8,500°F.
"Recent discoveries of water-rich Neptune-like exoplanets necessitate a thorough comprehension of the phase diagram of water under conditions akin to their planetary interiors," elucidated Gleason and her team in their January 2022 paper.
Phase diagram of H2O. Revised phase diagram for H2O including our data for BCC-structured ice (black circles) which shifts the FCC boundary to higher pressures in line with theoretical predictions. Blue dashed line is the Neptunian isentrope. (CREDIT: Nature)
Even though the extreme conditions existed for a brief moment, X-ray diffraction managed to capture the crystal structure of this dense ice. Astonishingly, the results pointed towards a new phase—distinct from the superionic ice detected in 2019. This newly identified superionic ice, aptly named Ice XIX, boasts a body-centered cubic structure and heightened conductivity compared to its 2019 counterpart, Ice XVIII.
Why is conductivity in this context so crucial?
Charged particles in motion generate magnetic fields, as explained by dynamo theory. This principle elucidates the genesis of magnetic fields in Earth's mantle and other celestial entities. Consequently, if a Neptune-like ice giant contained more solid ice and less liquid, the nature of its magnetic field would differ.
What's more compelling is the proposition that within a planet like Neptune, two superionic layers with varying conductivity might coexist. Such a structure would lead to the outer liquid layer's magnetic field interacting distinctively with each, yielding even more unconventional results.
Gleason and her team postulate that the heightened conductivity of an Ice XIX-like superionic layer could be the key to the generation of the unconventional, multipolar magnetic fields observed in Uranus and Neptune.
Such a conclusion, if verified, would bring a gratifying closure to the mysteries observed over 30 years ago when NASA's Voyager II space probe, dispatched in 1977, journeyed past our Solar System's enigmatic ice giants, recording their peculiar magnetic fields.
As we stand at the frontier of this discovery, one thing is clear: the universe remains a trove of mysteries, continually challenging and expanding our understanding. And while the nuances of superionic ice have only just begun to be understood, its implications for our comprehension of the cosmos are vast.
For more environmental good news stories check out our Green Impact section at The Brighter Side of News.
Note: Materials provided above by The Brighter Side of News. Content may be edited for style and length.
Like these kind of feel good stories? Get the Brighter Side of News' newsletter.
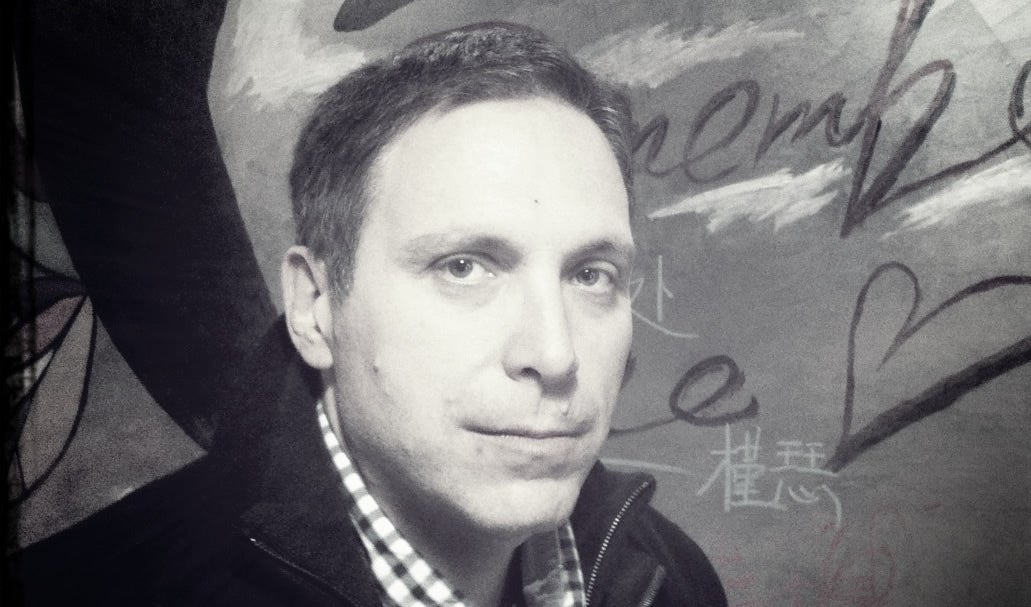