AI develops life-saving snake venom treatment – helping two million people per year
Breakthrough in computational protein design offers safer, cost-effective treatments for snakebites, neutralizing toxins with precision and consistency.
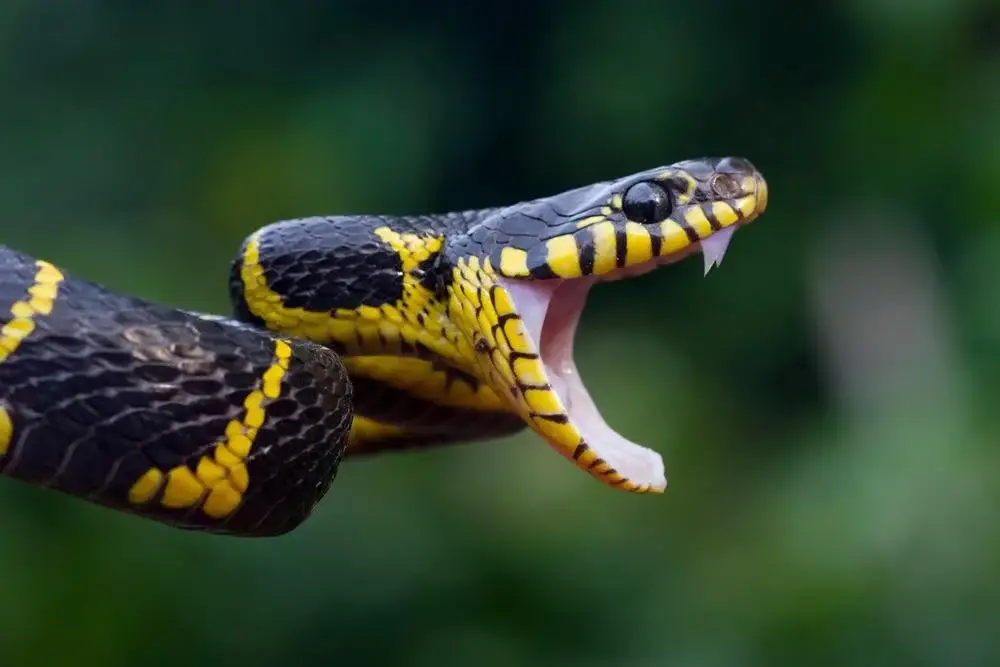
A deep learning breakthrough in computational protein design could transform snakebite treatments, offering safer, affordable, and highly effective solutions. (CREDIT: Shutterstock)
Each year, over two million people fall victim to snakebites, resulting in more than 100,000 fatalities and 300,000 severe disabilities, according to the World Health Organization.
These alarming statistics underline the global burden of snakebite envenoming, a neglected tropical disease that disproportionately affects low-resource regions like sub-Saharan Africa, South Asia, Papua New Guinea, and Latin America.
Traditional treatments, while life-saving, come with high costs, limited effectiveness against certain venom components, and significant side effects.
Conventional antivenoms rely on polyclonal antibodies derived from the plasma of immunized animals. While these therapies have saved countless lives, they are fraught with challenges. Production is expensive, requiring cold-chain storage, which is often inaccessible in remote areas.
Furthermore, these treatments are less effective against specific venom components, such as three-finger toxins (3FTxs) found in elapid snake venoms. These toxins, which can disrupt nerve-muscle communication, lead to paralysis and even death. Efforts to improve antivenoms have been slow and labor-intensive, leaving a pressing need for innovative solutions.
The reliance on animal immunization for antivenom production also introduces variability between batches, impacting consistency and effectiveness. Additionally, the size of traditional antibodies limits their ability to penetrate tissue, delaying their action against venom components. These shortcomings highlight the critical need for novel approaches that can address these limitations while reducing costs and improving accessibility.
A team of researchers from the UW Medicine Institute for Protein Design and the Technical University of Denmark have made significant strides using computational biology to address these challenges. Led by Susana Vazquez Torres, the team employed deep learning to design proteins capable of neutralizing 3FTxs. This approach represents a transformative shift from traditional methods, offering the potential for safer, more effective, and affordable antivenom therapies.
Using RFdiffusion, a deep learning-based method, the researchers designed proteins that bind to short-chain and long-chain α-neurotoxins and cytotoxins—key components of 3FTxs. These proteins were synthesized through recombinant DNA technology, enabling consistent production and avoiding the variability associated with animal-derived antibodies. Computational tools allowed for precise modeling of protein structures, ensuring high specificity and binding affinity.
This computational approach also significantly accelerates the discovery process. By bypassing the need for extensive toxin isolation or animal immunization, researchers can focus on designing highly targeted proteins. This streamlining reduces costs and minimizes the environmental and ethical concerns associated with traditional antivenom production.
The team’s efforts focused on creating binders for α-neurotoxins, which are known for their intricate structures stabilized by disulfide bridges. These toxins, present in many elapid snake venoms, disrupt nicotinic acetylcholine receptors (nAChRs), leading to severe neurotoxicity. By leveraging RFdiffusion and ProteinMPNN, the researchers generated designs with remarkable binding affinities and thermal stability.
Related Stories
One standout design, LNG, demonstrated a binding affinity of 1.9 nM against α-cobratoxin, as measured by surface plasmon resonance. The protein exhibited high thermal stability, with a melting temperature exceeding 95°C. Structural analysis through X-ray crystallography confirmed that LNG’s binding mode matched the computational design, showcasing the precision of the method.
The LNG design interacts with critical regions of the α-cobratoxin, blocking its ability to bind to nAChRs. This mechanism of action is crucial for preventing the neurotoxin’s harmful effects. The precise fit of LNG to its target highlights the potential of computational design to create highly effective therapeutic proteins.
Cytotoxins, another major group within 3FTxs, pose unique challenges due to their ability to destabilize cell membranes. These toxins cause significant local tissue damage, leading to complications like limb deformity and amputation.
The researchers targeted the consensus sequence of 86 different snake cytotoxins, creating designs that interact directly with the three-finger loops of these toxins. Among the 55 protein designs expressed, CYTX emerged as a promising candidate, showing high solubility and effective neutralization of venom from Naja pallida and Naja nigricollis. CYTX achieved a dissociation constant of 271 nM and exhibited thermal stability with a melting temperature of 61°C.
Further optimization led to the development of CYTX_B10, a variant with enhanced stability. X-ray crystallography revealed extensive interactions between CYTX_B10 and the cytotoxin, underscoring the potential of RFdiffusion to design highly specific and effective antivenom proteins. These interactions involve hydrogen bonds and electrostatic forces, which enhance the protein’s binding strength and neutralizing capacity.
The design of CYTX_B10 also demonstrates the flexibility of computational methods. By introducing targeted modifications, the researchers improved the protein’s thermal stability and solubility, ensuring its suitability for therapeutic use. This adaptability is a significant advantage over traditional antibody-based approaches.
The designed proteins underwent rigorous testing to validate their neutralizing capabilities. In patch-clamp experiments using human-derived muscle cells, designs like LNG and SHRT achieved complete neutralization of α-neurotoxins at a 1:1 molar ratio. These results outperformed existing nanobody-based treatments, highlighting the efficacy of the new designs.
When tested in vivo, the proteins protected mice from lethal doses of neurotoxins, demonstrating their potential for real-world applications. Their small size and high stability could allow for rapid tissue penetration, minimizing local damage and enhancing therapeutic outcomes. This rapid action is particularly critical in snakebite cases, where delays in treatment can lead to severe complications.
The researchers also emphasized the importance of designing proteins with broad neutralization capabilities. By targeting consensus sequences and conserved regions of toxins, the team created binders that are effective against multiple venom types. This broad-spectrum approach could significantly improve the versatility and accessibility of antivenom therapies.
This research exemplifies how computational protein design can revolutionize the development of therapeutics for neglected diseases. By enabling the production of consistent, cost-effective proteins, this approach addresses the limitations of traditional antivenoms. Moreover, the designed proteins’ high stability and broad neutralization capabilities pave the way for next-generation treatments that could be accessible to resource-limited regions.
“Computational design methodology could substantially reduce the costs and resource requirements for the development of therapies for neglected tropical diseases,” the researchers noted. Beyond snakebites, these methods hold promise for discovering antidotes and treatments for other diseases.
The study, published in Nature, was led by Susana Vazquez Torres, with senior researchers Timothy J. Perkins and David Baker. Their groundbreaking work underscores the transformative potential of combining computational biology with experimental validation to tackle global health challenges.
The researchers are optimistic about the future applications of their work. They envision using computational design to address other venomous bites and stings, as well as developing treatments for diseases with limited therapeutic options. By leveraging deep learning and advanced modeling techniques, scientists can accelerate the discovery of novel drugs and antidotes.
The University of Washington has submitted a provisional U.S. patent application for the design and composition of the proteins created in this study. This step highlights the commercial and clinical potential of the research, ensuring that the innovations can be developed into accessible treatments.
Note: Materials provided above by The Brighter Side of News. Content may be edited for style and length.
Like these kind of feel good stories? Get The Brighter Side of News' newsletter.