The human brain is taking micro-naps throughout the day
Scientists have found that sleep can be detected by patterns of neuronal activity, showing new ways to study basic brain wave patterns.
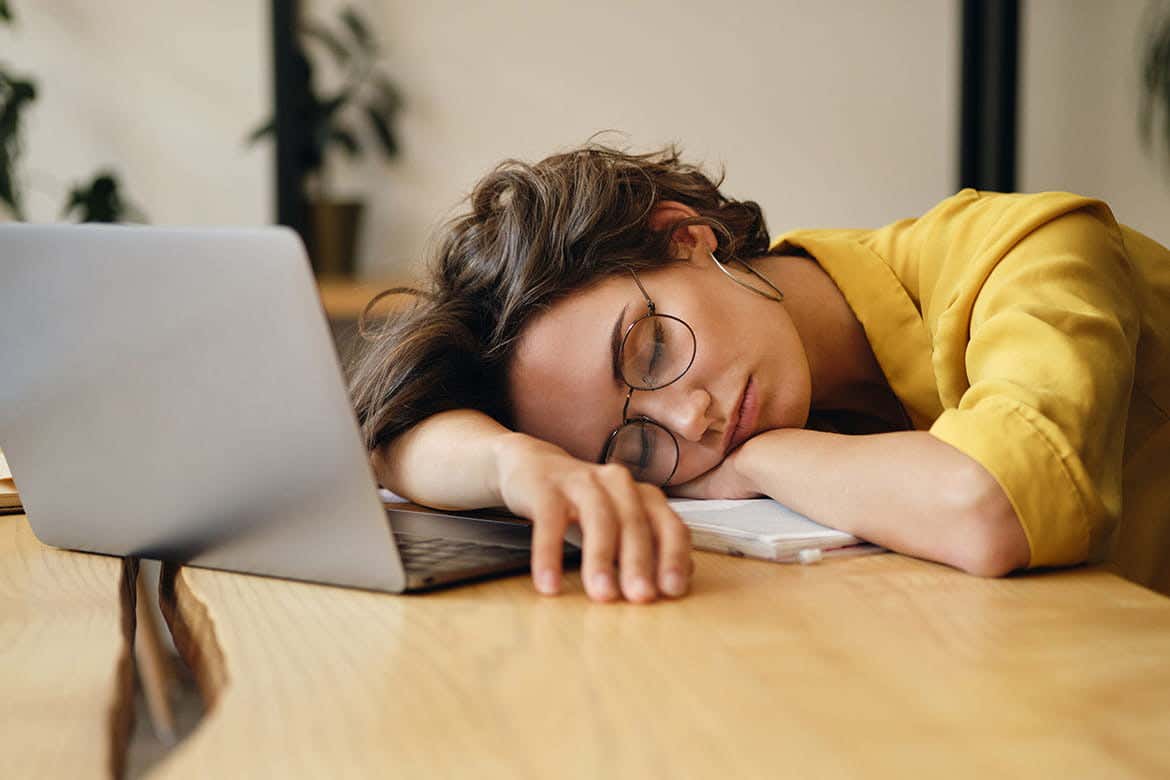
Sleep can be detected by patterns of neuronal activity that are just milliseconds long, a thousand times shorter than a second. (CREDIT: Creative Commons)
Sleep and wakefulness define the boundaries of our daily lives, serving as two distinct states of being. Scientists have traditionally measured the differences between these states by observing brain waves. Sleep is typically characterized by slow, long-lasting waves that travel across the entire brain.
However, new research has unveiled a groundbreaking discovery: sleep can be detected by patterns of neuronal activity that are just milliseconds long, a thousand times shorter than a second. This finding opens up new avenues for studying and understanding the basic brain wave patterns that govern consciousness.
This study, published in Nature Neuroscience, resulted from a collaboration between Assistant Professor Keith Hengen of Washington University in St. Louis and Distinguished Professor David Haussler of UC Santa Cruz. Ph.D. students David Parks (UCSC) and Aidan Schneider (WashU) carried out the research over four years. They trained a neural network to analyze massive amounts of brain wave data, uncovering high-frequency patterns that challenge long-held beliefs about the neurological basis of sleep and wakefulness.
“With powerful tools and new computational methods, there’s so much to be gained by challenging our most basic assumptions and revisiting the question of ‘what is a state?’” Hengen remarked. “Sleep or wake is the single greatest determinant of your behavior, and then everything else falls out from there. So if we don't understand what sleep and wake actually are, it seems like we’ve missed the boat.”
Haussler added, “It was surprising to us as scientists to find that different parts of our brains actually take little naps when the rest of the brain is awake, although many people may have already suspected this in their spouse.”
Neuroscientists study the brain by recording electrical signals, known as electrophysiology data, to observe voltage waves as they crest and fall. Mixed into these waves are the spike patterns of individual neurons. The researchers worked with data from mice in the Hengen Lab, where the animals were equipped with lightweight headsets that recorded brain activity from ten different regions for months at a time. This setup tracked voltage from small groups of neurons with microsecond precision, creating petabytes of data.
David Parks led the effort to feed this raw data into an artificial neural network, which can find highly complex patterns. This process differentiated sleep and wake data, uncovering patterns that human observation might have missed. The collaboration with UC San Diego’s compute infrastructure enabled the team to work with this massive amount of data, similar to what large companies like Google or Facebook might use.
Parks began feeding smaller and smaller chunks of data into the neural network to predict if the brain was asleep or awake. The model could differentiate between sleep and wake from just milliseconds of brain activity data, a finding that shocked the research team. This showed that the model couldn’t have been relying on the slow-moving waves traditionally associated with sleep.
“We're seeing information at a level of detail that’s unprecedented,” Haussler said. “The previous feeling was that nothing would be found there, that all the relevant information was in the slower frequency waves. This paper says, if you ignore the conventional measurements and look at the details of the high-frequency measurement over just a thousandth of a second, there is enough there to tell if the tissue is asleep or not. This tells us that there is something going on at a very fast scale — that's a new hint to what might be going on in sleep.”
Hengen initially believed that Parks and Schneider had missed something, as their results contradicted foundational concepts in neuroscience. He challenged Parks to produce more evidence to support their findings. “This challenged me to ask myself ‘to what extent are my beliefs based on evidence, and what evidence would I need to see to overturn those beliefs?’” Hengen said. “It was a really interesting process as a scientist to have my students tear down these towers brick by brick, and for me to have to be okay with that.”
To understand what patterns the model was learning from, Parks stripped away layers of temporal and spatial information. Eventually, they examined chunks of brain data just a millisecond long at the highest frequencies of brain voltage fluctuations. “We'd taken out all the information that neuroscience has used to understand, define, and analyze sleep for the last century, and we asked ‘Can the model still learn under these conditions?’” Parks explained. “This allowed us to look into signals we haven’t understood before.”
The researchers found that the hyper-fast pattern of activity between just a few neurons was the fundamental element of sleep that the model detected. Such patterns cannot be explained by traditional, slow and widespread waves. They hypothesize that slow-moving waves may coordinate fast, local patterns of activity, but concluded that fast patterns are closer to the true essence of sleep.
Observing flickers of activity, where small regions of the brain momentarily “wake up” while the rest of the brain remains asleep (and vice versa), added another layer of complexity to their findings. These “flickers” challenge established rules about the brain’s sleep cycle and could have significant implications for understanding neurodevelopmental and neurodegenerative diseases, both of which are associated with sleep dysregulation.
“There’s a natural hypothesis there; let’s say a small part of your brain slips into sleep while you’re awake — does that mean your behavior suddenly looks like you’re asleep? We started to see that that was often the case,” Schneider said. Observing mice, they found that when a brain region flickered to sleep, the mouse would pause, almost like zoning out. A flicker during sleep would cause the mouse to twitch.
Understanding these high-frequency patterns and flickers could help researchers study diseases and disorders more effectively. Hengen noted, “This gives us potentially a very, very sharp scalpel with which to cut into these questions of diseases and disorders. The more we understand fundamentally about what sleep and wake are, the more we can address pertinent clinical and disease-related problems.”
On a foundational level, this work advances our understanding of the brain's complexity, influencing behavior, emotion, and much more. The research highlights the need to continually question and refine our knowledge, using advanced tools and methods to uncover the brain’s many layers of intricacy.
Note: Materials provided above by the The Brighter Side of News. Content may be edited for style and length.
Like these kind of feel good stories? Get the Brighter Side of News' newsletter.
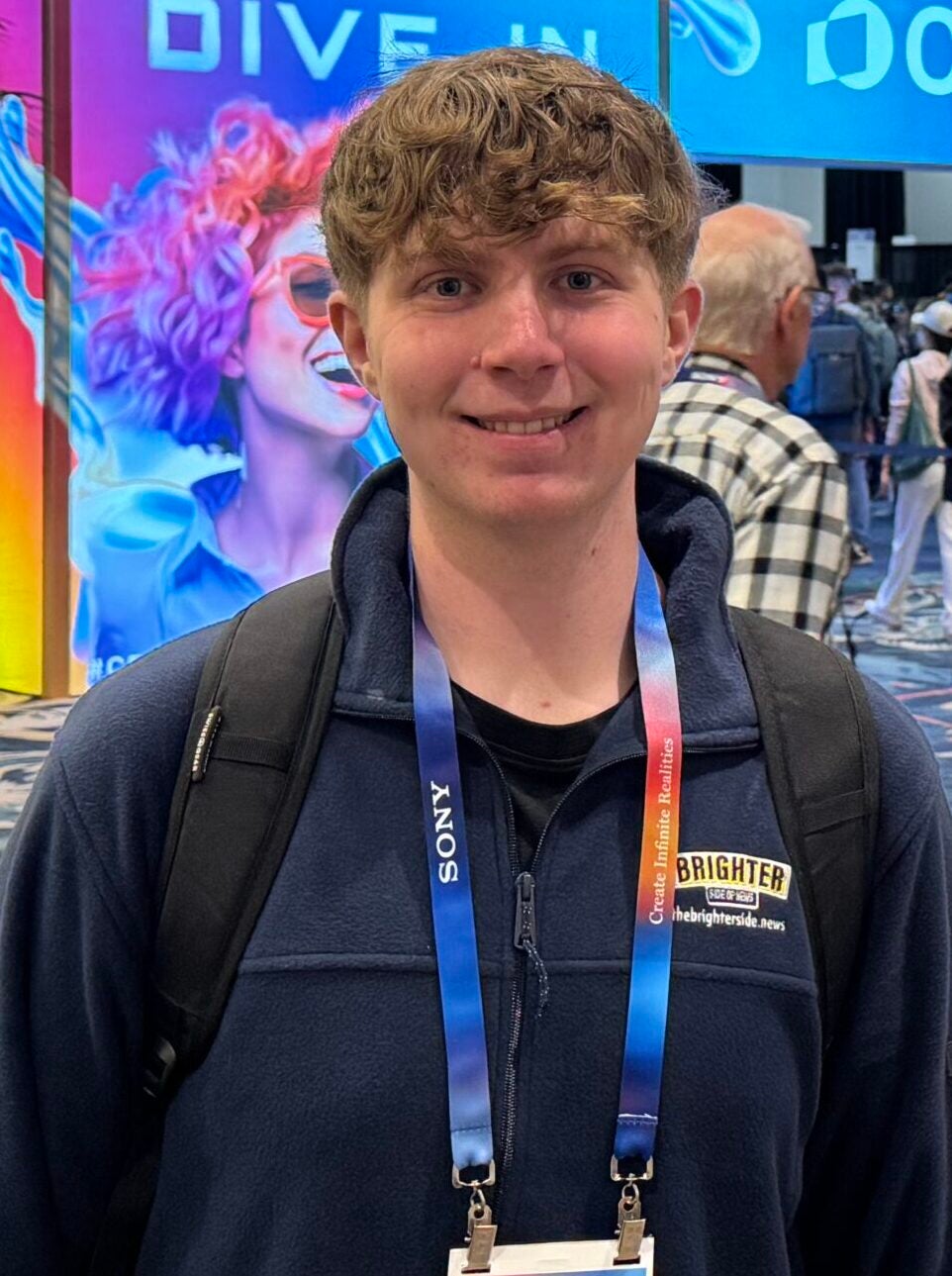
Joshua Shavit
Science & Technology Writer | AI and Robotics Reporter
Joshua Shavit is a Los Angeles-based science and technology writer with a passion for exploring the breakthroughs shaping the future. As a contributor to The Brighter Side of News, he focuses on positive and transformative advancements in AI, technology, physics, engineering, robotics and space science. Joshua is currently working towards a Bachelor of Science in Business Administration at the University of California, Berkeley. He combines his academic background with a talent for storytelling, making complex scientific discoveries engaging and accessible. His work highlights the innovators behind the ideas, bringing readers closer to the people driving progress.