New optical atomic clock is 1,000x more accurate than today’s standard
The way time is measured is on the edge of a historic upgrade through a new kind of atomic clock that uses light instead of microwaves.
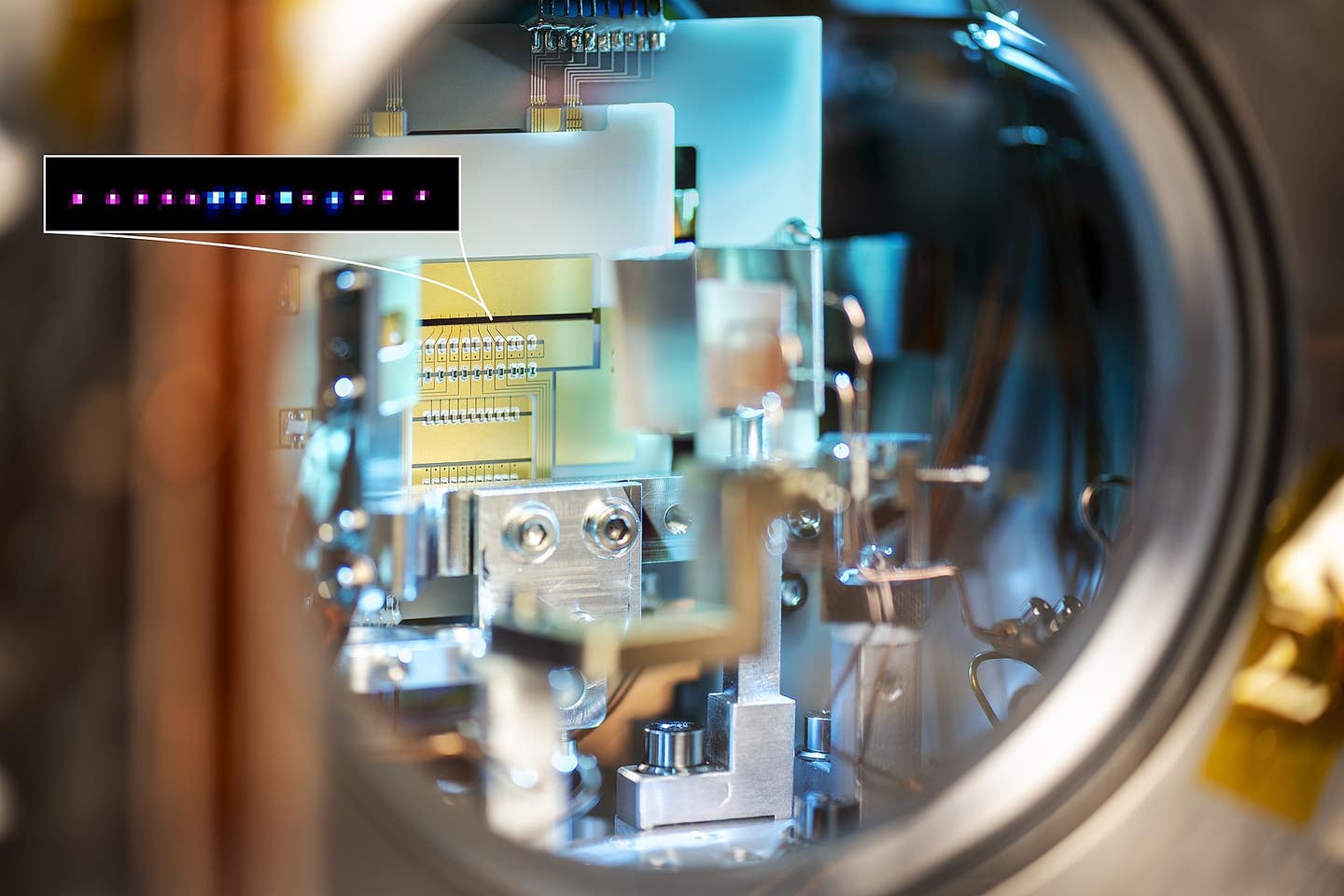
The ion trap of the new In+/Yb+-crystal clock in its vacuum chamber. The Ions are trapped in the gap that can be seen in the middle of the picture between the gold electrodes (target). A shown-up picture: a crystal from indium- (pink) and ytterbium (blue) ions. (CREDIT: PTB)
The way time is measured is on the edge of a historic upgrade. At the heart of this change is a new kind of atomic clock that uses light instead of microwaves. This shift means timekeeping could become 1,000 times more accurate than today's standards.
Researchers at the Physikalisch-Technische Bundesanstalt (PTB) in Germany have pushed atomic clocks into the future. Their latest device uses indium ions arranged in a linear crystal. It’s part of a long-term goal to redefine the second based on optical clocks. For now, that still depends on microwave transitions in caesium atoms, but this might change soon.
A faster tick: optical clocks vs. caesium clocks
Right now, the world defines one second based on the frequency of microwaves emitted by caesium atoms. But this method, though reliable, is starting to show its limits. Optical clocks “tick” at much higher frequencies—around 100,000 times faster than their caesium-based cousins. This higher frequency allows them to divide time into finer slices.
The precision of optical clocks has become so sharp that if one had been running since the Big Bang, it would be off by only a single second today. Still, optical clocks have been limited by the fact that most use just one ion to mark time. That makes them fragile and slow to average out noise, often requiring weeks—or even years—of data to match their potential.
PTB’s new clock solves this issue by using multiple ions at once. This multi-ion approach leads to faster and more reliable measurements. It also allows researchers to mix ion types, combining their strengths.
Crystals of time: building a better ion clock
The latest optical clock uses a chain of four ions: one indium ion that acts as the "clock" and three ytterbium ions that cool it down. These ions interact to form a crystal-like structure held in place by electric fields inside a vacuum. The setup is housed in a compact 3D chip ion trap. The chip supports several motional frequencies, with center-of-mass motions for ytterbium ions measured at about 822 kHz, 794 kHz, and 275 kHz.
Related Stories
This “Coulomb crystal” keeps the ions organized and isolated from outside forces. Since any disturbance can affect the laser’s frequency match with the atoms, researchers must be able to detect and correct even the tiniest shifts. PTB’s team developed a method to reorder the ions in the crystal to ensure consistent performance, using a system that tracks positions with binary strings—for example, "1011" means an indium ion is followed by three ytterbium ions.
To check and control the ion positions, the system uses a special imaging setup. It detects site-specific light emissions from both ion types—369.5 nm for ytterbium and 230.6 nm for indium. If needed, the system can “rescue” a disrupted crystal by cooling it, reforming its shape, or breaking apart molecules formed during background gas collisions.
Taming the laser: how the clock stays on beat
The clock’s laser must match the energy jump of the indium ions exactly. To do this, researchers use a stabilized Nd:YAG laser, operating at 946 nm. This laser is locked to a cryogenic silicon resonator, which keeps it from drifting. The light is then converted to the required 230.6 nm to excite the indium ions.
Each cycle of the clock has four parts: preparation, cooling, interrogation, and detection. In the first step, the system checks the crystal layout and ensures the ions are properly arranged. The indium ion is then pumped into a specific state. After that, ytterbium ions cool the system through Doppler cooling. A rectangular pulse, 150 milliseconds long, probes the clock transition in the indium ion. Finally, a detector checks if the indium ion made the expected jump.
If the crystal arrangement shifts, the data is discarded. Only results from well-prepared crystals are kept to ensure accuracy. This method not only boosts performance but makes the clock scalable. Researchers have shown it works with up to four ions, which paves the way for even more complex setups.
Results that redefine precision
This clock reached a fractional systematic uncertainty of just 2.5 × 10⁻¹⁸—an incredible level of accuracy. To put it in context, that’s equivalent to measuring a second so precisely that it would only drift by one second over 13.8 billion years.
The PTB team compared their new clock to two other optical clocks—one based on strontium atoms and the other on ytterbium ions. These comparisons had relative uncertainties of 4.2 × 10⁻¹⁷ and 4.4 × 10⁻¹⁸, respectively. That’s more than ten times better than previous measurements. These results support the clock’s reliability and make it a strong candidate for future international time standards.
The team also measured the absolute frequency of the indium transition, an important step toward redefining the SI second. Right now, that redefinition depends on building confidence in several optical clocks and confirming their consistency through global comparisons.
Jonas Keller, one of the physicists at PTB, emphasized the advantage of combining ion types: “We use indium ions as they have favorable properties to achieve high accuracy. For efficient cooling, ytterbium ions are added to the crystal.”
Group leader Tanja Mehlstäubler and her team worked to solve major challenges in designing this new system. Their work included finding new ways to position the ions and keep the crystal structure stable. Thanks to their success, the clock now reaches accuracy levels close to the 18th decimal place.
The future of timekeeping
With this design, optical ion clocks are no longer limited to slow measurements and single particles. They can scale up, speed up, and match or surpass any other clock in the world. This opens the door to new concepts like quantum many-body clocks or chained ensembles of atoms. It may even lead to technologies beyond just telling time—such as better GPS, more precise geophysical measurements, and tests of fundamental physics.
This work, published in Physical Review Letters, received support from the German Research Foundation (DFG) under the Quantum Frontiers Cluster of Excellence and the DQ-mat Collaborative Research Center. The results mark a turning point in precision timekeeping—and bring us closer to a future where time itself is measured by light.
Note: The article above provided above by The Brighter Side of News.
Like these kind of feel good stories? Get The Brighter Side of News' newsletter.
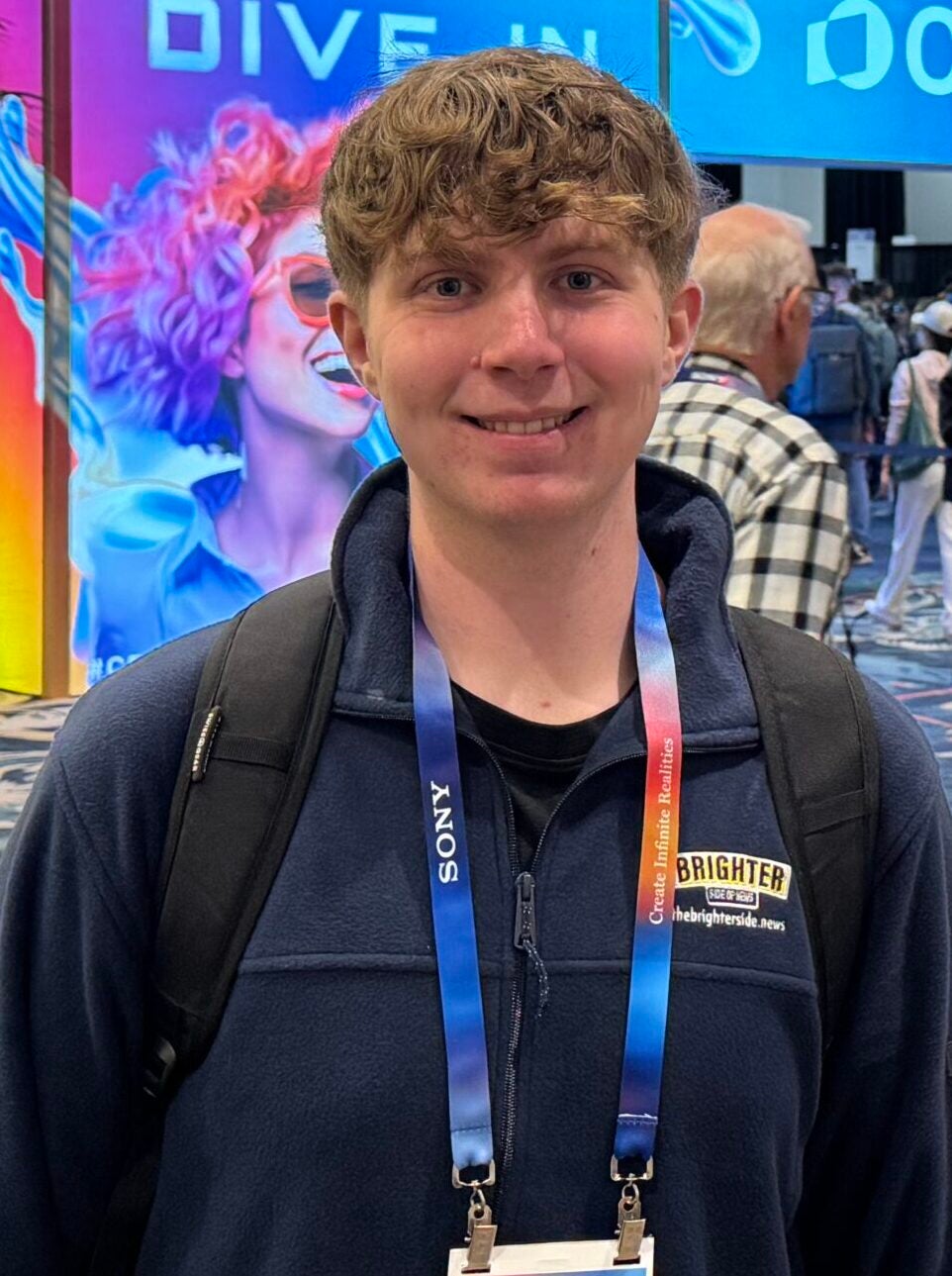
Joshua Shavit
Science & Technology Writer | AI and Robotics Reporter
Joshua Shavit is a Los Angeles-based science and technology writer with a passion for exploring the breakthroughs shaping the future. As a contributor to The Brighter Side of News, he focuses on positive and transformative advancements in AI, technology, physics, engineering, robotics and space science. Joshua is currently working towards a Bachelor of Science in Business Administration at the University of California, Berkeley. He combines his academic background with a talent for storytelling, making complex scientific discoveries engaging and accessible. His work highlights the innovators behind the ideas, bringing readers closer to the people driving progress.